1. Introduction
Peripheral nerve injury is one of the most common damages; many lead to permanent disabilities and neuropathic pain (
Sullivan, Dailey, Duncan, Abel, & Borlongan, 2016). The most severe of which is called neurotmesis that results in the loss of nerve trunk continuity, myelin sheath, and the surrounding connective tissues. If there is no gap between the cut endings of the nerve, or if the gap is short, the two nerve endings can be directly sutured, and the result of healing will depend on whether the nerve bundles at the cut endings to adhere to each other correctly (
Mafi, Hindocha, Dhital, & Saleh, 2012).
Although some drugs such as cyclosporine A and melatonin have useful effect for axonal regeneration and sprouting effect for peripheral nerve injury (
Roozbehi, Joghataie, Mehdizadeh, Mirzaei, & Delaviz, 2012;
Turgut & Kaplan, 2011), in the long gap of peripheral nerve injuries, diversion of axonal buds at the injury site is a significant problem that can influence nerve repair (
Panagopoulos, Megaloikonomos, & Mavrogenis, 2017). Therefore, this gap must be bridged so that the generated axons can be guided toward the distal stump and target organ. In these cases, the material used for bridging the gap can influence the result of the repairing process (
Panagopoulos et al., 2017).
Polyvinylidene fluoride channel as a conduit for bridging the peripheral nerves defects with nerve growth factor (NGF) and collagen gel could provide a favorable matrix for axonal regrowth (
Delaviz et al., 2011). The basal membrane could stimulate and create guidance channels for regrowth of axons toward the target tissue (
Kang, Hu, Wang, & Wang, 2015). It has been shown that muscle basal lamina grafts can be a guidance route for the injured axons toward the distal segment of the endoneurial tubes (
La Fleur, Underwood, Rappolee, & Werb, 1996).
Although nerve graft is considered a standard procedure, yet sacrificing a peripheral nerve is not an ideal option since it can lead to numbness in the receptor zone and may also cause the formation of neuromas and visible scars (
Daly, Yao, Zeugolis, Windebank, & Pandit, 2012). Therefore, it is better to find a suitable guidance channel at the site of the nerve gap that can serve as a favorable substrate and nutritional support that cause axonal growth and, consequently, functional recovery. The similarity between the coatings of skeletal muscle fiber with nerve fibers makes it possible to use autologous muscle grafts as a conduit in nerve repair (
Roganovic, Ilic, & Savic, 2007).
Clinical recovery and histological evidence of the axonal regeneration has been observed after muscle grafting of the nerve damage in leprosy (
de Blaquiere, Curtis, Pereira, & Turk, 1994). Epimysial of degenerated muscle graft as a tube can guide the regenerating nerve fiber to the distal end of the nerve defect (
Yang et al., 2013).
To create natural guidance channels that provide favorable conditions for axonal growth and prevent immune responses, it is recommended that a cellular skeletal muscle graft be used in the gap region (
Roganovic et al., 2007). Cellular constituents in the skeletal muscle tissue can be removed using various techniques, including initial treatment with alcohol, freezing, and thawing (
Roganovic et al., 2007). Exogenous growth factor, along with a nerve conduit, plays a vital role in neuronal survival and nerve regeneration (
Ma et al., 2016). It was found that nerve guidance channels containing collagen and laminin-containing gels have better regeneration capacity compared to those that contain only a saline medium (
Verdú et al., 2002). Moreover, various isoforms of laminin, as initial compounds of the basal lamina, are considered potent stimulants for axonal growth and repair (
Chen & Strickland, 2003). Therefore, in the present research, the effect of a cellular skeletomuscular graft with nerve growth factor and laminin are studied on axonal regeneration and functional recovery following sciatic nerve transection.
2. Methods
2.1. Animals and groups
This experimental research was done according to the guidelines of Iranian Syndicate for Application and Care of Animals and was approved by the Animal Research Ethics Committee of Yasuj University of Medical Sciences. Forty-two female albino rats of the Wistar strains (Weight: 200±250 g) were purchased from the animal house of the Shiraz University of Medical Sciences. The animals were housed under conditions of controlled temperature (22˚C±2˚C) with 12-h light/12-h dark cycle and food and water ad libitum. The animals were randomly assigned into three equal groups of 14: the muscle graft+NGF+laminin group, the muscle graft group, and the control group.
2.2. Surgical procedure and muscle autografts
The rats were anesthetized via intraperitoneal injection of ketamine (100 mg/kg) and xylazine (10 mg/kg). An incision was made on the posterior surface of the left thigh from the sciatic notch to the popliteal region, the muscles and fascia were pushed away, and the sciatic nerve was exposed. Under aseptic condition at mid-thigh level, 10 mm of the sciatic nerve was cut and removed.
A 12-mm narrow strip of the left gluteus superficialis muscle was removed in alignment with the lengths of the muscle fibers. The narrow strip was placed in liquid nitrogen for 5 minutes to freeze completely, then put in normal saline solution for more than 5 minutes at room temperature. Next, it was placed in sterile distilled water for 10 minutes so that the cytoplasm and cell membrane were removed due to the osmotic phenomenon (through leakage) from the muscle (
Glasby, Gschmeissner, Huang, De Souza, 1986). Following that, a surgical blade was used to trim the thawed muscle in the form of a square block (2×2×12 mm) under a stereomicroscope.
One millimeter of both stumps of the distal and proximal sciatic nerve was placed inside muscular graft, and the epimysium of the muscle was sutured to the epineurium of the sciatic nerve at the proximal and distal ends of the nerve with using absorbable suture 10/0 (Ethicon). In the muscle graft+NGF+laminin group, 100 ng of the nerve growth factor (NGF) (Sigma-Aldrich, USA) followed by (1.28 mg/mL) laminin (Sigma) were injected into the muscle graft (
Labrador, Butı́, & Navarro, 1998). In the muscle graft group, normal saline was injected into the muscle graft. The 10-mm sciatic nerve was transected and removed with the same procedure without any treatment in the control group.
2.3. Walking track analysis
Assessment of the functional recovery was done 1, 8, 15, 22, 29, and 60 days after the operation with the measurement of the Sciatic Functional Index (SFI) (
Bain, Mackinnon, & Hunter, 1989). The rats’ hind limbs were dipped in Iranian ink and permitted to walk in a wooden box (70×30×20) that covered with a sheet of white paper to record the footprints. The SFI was calculated on the operated and healthy legs of the rats based on the formula introduced by Bain et al. (
Bain et al., 1989):
SFI=−38.3 (EPL-NPL)/NPL+109.5(ETS-NTS)/NTS+13.3(EIT-NIT)/NIT−8.8
where the Print Length (PL), toe spread from the first to the fifth Toe (Ts), and the Intermediary Toe spread (IT) from the second to the fourth toe were measured on the experimental (EPL, ETS, and EIT) and normal sides (NPL, NTS, and NIT). If SFI is equal to or less than -100, the motor function has been completely lost, values between -100 and -10 indicate an improving trend in the motor function, and those between -10 and +10 represent a normal function of the legs.
2.4. Retrograde tracing of spinal motor neurons
Sixty days after treatment, eight rats from each group were anesthetized, and the gastrocnemius muscles on the left side were exposed. 1, 1-َDioctadecyl-3, 3, 3,َ 3 –tetramethylindocarbocyanine Perchlorate (DiI) (Molecular Probes, Leiden, The Netherlands; cat. No, D-282) was used for labeling the spinal motor neurons and evaluation of the axonal regrowth. With using 10-μL Hamilton syringe, 8-9 μL of DiI tracer in 170 mg/mL DMSO was diluted 1:10 in saline and injected into 5 different locations in the bulk of the gastrocnemius muscle (
Madison & Robinson, 2014). Ten days after application, the animals were perfused, and their spinal cord segment (L4-L6) was dissected out. Each spinal segment was serially sectioned into 50-μm horizontal sections on a freezing microtome (Leica cryostat). The labeled spinal motor neurons were counted using fluorescent microscopy (Olympus Ax70). As previously described, in each spinal segment, the number of labeled motor neurons of each section summed up together to give the total number of motor neurons for each rat (
Catapano et al., 2016).
2.5. Histological study
After 60 days post-treatment, 6 rats from each group were anesthetized with double doses of ketamine (200 mg/kg) and xylazine (20 mg/kg) and perfused for histological studies. For counting of myelinated nerve fibers at the grafted region, 12 mm of the sciatic nerve with the center of the transplanted area was cut and removed. This segment was divided into three equal 4-mm parts of proximal, middle, and distal. The proximal parts of each segment were marked to distinguish the distal end from the proximal one. For counting and morphological study of the spinal motor neurons that participated in the sciatic nerve, 5 mm of the L4-L6 spinal cord segment was cut and removed. The spinal cord and each segment of the sciatic nerve were cut into the 50 µm and 5 µm segments, respectively (Leica cryostat, CM 3000).
The number of myelinated nerve fibers and motor neurons in each segment of the spinal cord or sciatic nerve was counted under a microscope (Olympus Bx51). The images of the section were taken with a digital camera (DP 11) connected to the microscope. As previously described (
Takami et al., 2002), the number of nerve fibers or neurons of each section was summed up to obtain the final number of nerve fiber or neuron in a 5-mm long spinal cord or 4-mm sciatic nerve segment in each rat.
2.6. Statistical analysis
All statistical analyses were performed in SPSS 18.0 (SPSS Inc., USA). One-way ANOVA followed by post hoc Tukey’s were used for data analysis. All data are expressed as Mean±SD. P<0.05 was considered to be statistically significant.
3. Results
3.1. Gait analysis
Assessment of the functional recovery was conducted by a researcher who was blind to animal grouping. One day after the operation, the rats dragged their feet with foot drop, and toe adduction in all three groups. Based on Table 1, gait analysis on day 1 and 8 in the different groups indicates that function of the sciatic nerve has been lost, and 1-way ANOVA analyses demonstrate no significant differences between the three groups on the injured foot (P>0.05). The ability to walk on the operated legs in the muscle graft+NGF+laminin and muscle graft groups improved on day 29, and the mean value of SFI increased significantly in these two groups compared to the control group (P<0.05) (Figure 1).
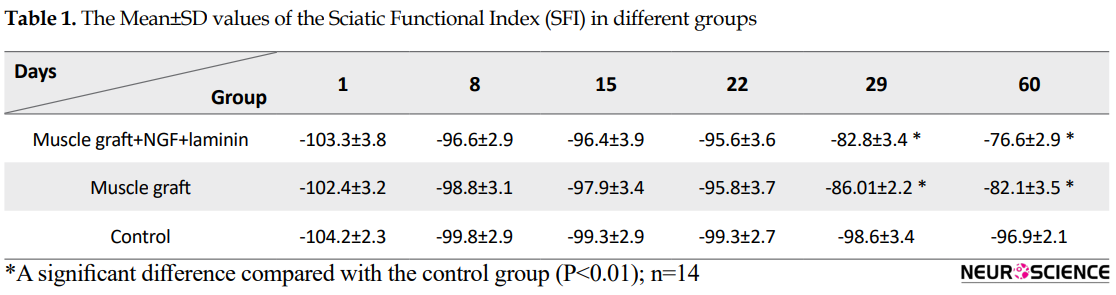
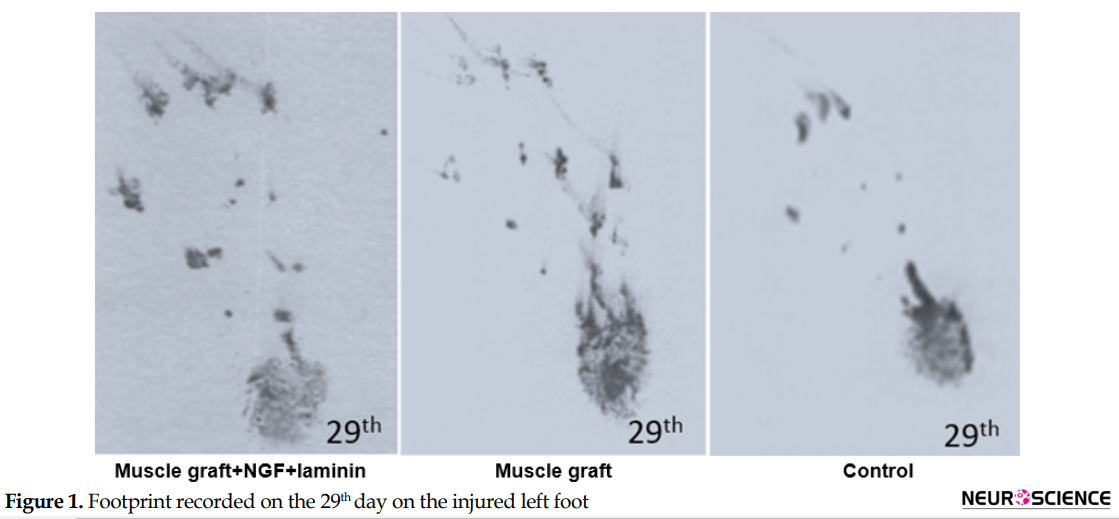
Sixty days after the operation, the treated group with muscle graft+NGF+laminin on the left hind limb showed toe-spreading and better footprints walking tracks compared to the other two groups. Statistical analysis on the 60th day indicated that the Mean±SD SFI increased significantly in the muscle graft +NGF+laminin (-76.6±2.9) and muscle graft (-82.1±3.5) groups compared to the control group (-96.9±2.1). Although improvement of functional motor movement in the muscle graft+NGF+laminin group increased compared to the muscle graft group, this difference was not statistically significant. It must be mentioned that one day before the operation, the normal gait was recorded in all three groups and hind limb toes were fully spread, and there were no significant differences between the groups.
3.2. Retrograde DiI labeled neuron
DiI positive nerve cells were observed in the perikaryon of the spinal cells through the axonal regrowth 70 days after treatment (Figure 2). DiI tracer in the perikaryon of the motor neuron in the muscle graft+NGF+laminin and muscle graft groups were seen more and more specific than those in the control group (Figure 2). The Mean±SD number of DiI labeled motor neurons in the spinal segment of the L4-L6 in the muscle graft+NGF+laminin, and muscle graft groups were 78.6±3.1 and 61.3±6.1, respectively. One-way ANOVA indicated that there were significant differences compared to the control group (25.2±2.2) (P<0.001) (Figure 3).

3.3. Histological studies
Histological assessment of the distal segment in the transplanted groups showed that axons could grow and reached the distal part (Figure 4). The thicknesses of myelin of the regenerating axons increased in the muscle graft+NGF+laminin and muscle graft groups compared to that in the control rats (Figure 4)..
The Mean±SD number of myelinated nerve fibers in the proximal segment in the muscle graft+NGF+laminin (3353±34.2) and muscle graft (3382±42.6) groups increased compared to that in the control group (3216±53.1) (Table 2). One-way ANOVA analysis showed no difference in the proximal segment between the groups (P>0.05). The Mean±SD number of myelinated nerve fibers in the middle segments in the muscle graft +NGF + laminin (343±11.1) and muscle graft (312±15.9) groups increased significantly compared to that in the control group (200±18.3) (P<0.05). The Mean±SD number of myelinated nerve fibers in the distal segments in the muscle graft+NGF+laminin (18.2±1.6) and muscle graft (14.6±2.1) groups increased significantly compared to that in the control group (6.3±3.4).Sciatic nerve transection reduced the number of motor neurons in the anterior horn of the injured side compared to that in the healthy side (Figure 5).

In each group, the mean number of spinal motor neurons decreased significantly in the left side of the L4-L6 spinal cord segment (The fiber of these neurons were transected in the left sciatic nerve) compared to the right side (P>0.05). The Mean±SD number of the motor neurons in the left side of the spinal segment in the muscle graft+NGF+laminin (475.5±6.1) and muscle graft (403.2±3.8) groups increased significantly compared to that in the control group (219.6±2.2) (Table 3). Although the number of motor neurons in the left side of the spinal segment increased in the muscle graft+NGF+laminin group compared to the muscle graft group, this increase was not significant (P>0.05).
4. Discussion
Peripheral nerve injury is a major problem that annually affects millions of people in the world, and most cases require surgical nerve repair (
Perretta & Green, 2017). A grafting procedure is necessary to improve the functional recovery when a long segment of the nerve is lost (
Mohammadi, Delaviz, Mohammadi, Delaviz, & Rad, 2016). When there is a large gap between the nerve endings, a suitable anatomical conduit is necessary so that the axons in the proximal segment can be guided into the endoneurial tubes of the distal segment and restore the function of the target organ (
Iijima, Ajiki, Murayama, & Takeshita, 2016).
To improve and accelerate nerve regrowth, different conduits, including olfactory mucosa, adult Schwann cells, or amniotic membrane with betamethasone were used in experimental studies (
Delaviz et al., 2008;
Hedayatpour et al., 2007;
Sadraie et al., 2016;
Zarinfard, Tadjalli, Razavi, & Kazemi, 2016). Among the various attempts, degenerated skeletal muscle autografts could recover the foot and hand sensation in patients with leprosy (
Pereira, Bowden, Narayanakumar, & Gschmeissner, 1996). This study confirms the results of other studies that showed the repair of sciatic nerve could enhance the survival of the spinal ganglion cells (
Atlasi, Mehdizadeh, Bahadori, & Joghataei, 2009).
Because of the similarity between the tubular matrix of skeletal muscles (the basal membrane) and the endoneurial tubes of a damaged nerve, it is possible to use autologous muscle grafts as a favorable biological conduit for nerve repair (
Kang et al., 2015). Therefore, the sheaths of skeletal muscle were employed as a guidance channel in repairing of nerve injury in the clinical and experimental research (
Meek, Varejão, & Geuna, 2004;
Pereira et al., 1996;
Sanes, 2003).
Gait analysis of this study indicated that motor function improved in the groups with degenerated muscle grafts compared to the control group. It has been shown that axonal regeneration could grow from the skeletal muscle grafts and reach the target muscle, and innervate the muscle fibers to improve the motor function (
Glasby, Gschmeissner, Huang, & De Souza, 1986). Our study results confirm the Glasby et al. study that demonstrated the use of non-neural autografts and skeletal muscle fibers with parallel array of the nerve fiber could create a matrix of basal membrane tubes that were anatomically and chemically similar to peripheral nerves sheet (
Glasby, et al., 1986).
Moreover, Norris et al. showed that the basal membrane tubes derived from skeletal muscles had sufficient diameter to match the largest nerve fibers (
Norris, Glasby, Gattuso, & Bowden, 1988). Basal membranes of muscle fibers were in the form of long cylinders lying parallel to each other and provide a suitable substrate for the growing axons (
Houštava, Dubový, Haninec, & Grim, 1999). The axons regrowth faster when the conduit matrix of the degenerating muscle is coaxial with the nerve fiber (
Glasby, Gschmeissner, Hitchcock, & Huang, 1986;
Glasby et al., 1986). Degenerated muscle grafts for nerve repair in primates guide the myelination of the nerve in the graft region and axonal regeneration to the distal nerve segment with the typical electrophysiological results (
Glasby, Gschmeissner, & Hitchcock, 1986).
In the present research, functional recovery and axonal regeneration improved in the muscle graft +NGF+laminin group compared to the muscle grafts and the control groups. NGF is essential for the survival of the neuron, synaptic development, axonal regrowth, and functional recovery in the central and peripheral nervous system (
Mesentier-Louro et al., 2017). The enriching of the conduit nerve tube with NGF increases the odds of axonal regeneration of a 10-mm long gap of the rat sciatic nerve (
Mesentier-Louro et al., 2017). Nerve growth factor by up-regulating p75NTR expression in Schwann cells could stimulate the nerve regrowth in the Wistar rats (
Ma, Peng, Wu, Wu, & Gao, 2013). Furthermore, the favorite microenvironment such as laminin and collagen can provide an appropriate matrix for axon regrowth (
Cao et al., 2011).
Laminin has biological activities such as neurite-promoting activity and can create a favorable microenvironment for neurotic regeneration in vitro (
Manthorpe et al., 1983). Nerve conduction tube filling with substrates such as laminin, fibronectin, and NGF provides a favorable condition for nerve regrowth and cell migration
(Glasgow et al., 2016). Suitable concentrations of laminin and growth factors are essential for nerve repair (
Valentini, Aebischer, Winn, & Galletti, 1987). The high concentration of laminin inhibits the diffusion of growth factors and act as a barrier for axonal regeneration (
Valentini et al., 1987).
Our result confirmed the Labrador et al. study results that showed a low concentration of laminin or collagen could provide better axonal regrowth (
Labrador et al., 1998). Laminin-8 and Laminin-2 play an vital role in the development of nerve growth and nerve regeneration after injury (
Wallquist et al., 2002). Laminin as a substrate affects Schwann cell migration during nerve repair and has effective role for axonal regeneration in a cellular nerve allograft (
Heermann & Schwab, 2013).
Furthermore, laminin with growth factors has a significant role for axonal regeneration in the long gap (20 mm) in the experimental study and NGF increase nerve regrowth and myelin formation (
Barbon et al., 2016;
Dodla & Bellamkonda, 2008). Our result confirmed Pu et al. study results that showed enriching of the conduit nerve tube with NGF increased the likelihood of axonal regeneration of a 10-mm long gap of the rat sciatic nerve (
Meek, 2000). Biological conduit such as combined muscle and vein could reconstruct the digital nerve defect up to 4 cm in patients (
Battiston, Geuna, Ferrero, & Tos, 2005). Although many studies do not use an intact group in their research, we think that one limitation of this study was the lack of an intact group that results can be compared with them.
No single drug or particular approach is available for the favorable and sound treatment of peripheral nerve injury. When a long part of nerve continuity is lost, one technique alone cannot provide adequate treatment for nerve repair and combined therapy with conduit tube might achieve better outcomes. Results of this study demonstrated that using biological tubulization such as degenerated skeletal muscle autografts with NGF and laminin could provide a suitable scaffold for bridging a nerve defect. This method is easy to use, less expensive, and without inflammatory responses or complications.
Ethical Considerations
Compliance with ethical guidelines
All the experiments were performed in compliance with the National Institutes of Health Guide for the Laboratory Animals Use and Care and approved by the Ethics and Research Committee of Yasuj University of Medical Sciences, Yasuj, Iran.
Funding
This study was a research project approved by the Research Deputy of Yasuj University of Medical Sciences.
Authors' contributions
Conceived, collected the data: Mehrzad Jafari; Designed and implemented the study protocol, interpreted the data and drafted the manuscript: Hamdollah Delaviz; Collected of the data and drafted the manuscript: Somayeh Torabi; Participated in the design of the study, drafted the manuscript and performed the statistical analysis: Jamshid Mahammadi; Conceived of the study and performed the statistical analysis: Izadpanah Gheitasi.
Conflict of interest
The authors declared no conflict of interest.
Acknowledgments
We want to express our gratitude to the personnel at the Cellular and Molecular Research Center of Yasuj for their help in carrying out this research.
References
- Atlasi, M. A., Mehdizadeh, M., Bahadori, M. H., & Joghataei, M. T. (2009). Morphological identification of cell death in dorsal root ganglion neurons following peripheral nerve injury and repair in adult rat. Iranian Biomedical Journal, 13(2), 65-72. [PMID]
- Bain, J. R., Mackinnon, S. E., & Hunter, D. A. (1989). Functional evaluation of complete sciatic, peroneal, and posterior tibial nerve lesions in the rat. Plastic and Reconstructive Surgery, 83(1), 129-38. [DOI:10.1097/00006534-198901000-00024] [PMID]
- Barbon, S., Stocco, E., Negro, A., Dalzoppo, D., Borgio, L., Rajendran, S., et al. (2016). In vitro assessment of TAT-Ciliary neurotrophic factor therapeutic potential for peripheral nerve regeneration. Toxicology and Applied Pharmacology, 309, 121-8. [DOI:10.1016/j.taap.2016.09.001] [PMID]
- Battiston, B., Geuna, S., Ferrero, M., & Tos, P. (2005). Nerve repair by means of tubulization: Literature review and personal clinical experience comparing biological and synthetic conduits for sensory nerve repair. Microsurgery, 25(4), 258-67. [DOI:10.1002/micr.20127] [PMID]
- Cao, J., Sun, C., Zhao, H., Xiao, Z., Chen, B., Gao, J., et al. (2011). The use of laminin modified linear ordered collagen scaffolds loaded with laminin-binding ciliary neurotrophic factor for sciatic nerve regeneration in rats. Biomaterials, 32(16), 3939-48. [DOI:10.1016/j.biomaterials.2011.02.020] [PMID]
- Catapano, J., Willand, M. P., Zhang, J. J., Scholl, D., Gordon, T., & Borschel, G. H. (2016). Retrograde labeling of regenerating motor and sensory neurons using silicone caps. Journal of Neuroscience Methods, 259, 122-8. [DOI:10.1016/j.jneumeth.2015.11.020] [PMID]
- Chen, Z. L., & Strickland, S. (2003). Laminin γ1 is critical for Schwann cell differentiation, axon myelination, and regeneration in the peripheral nerve. The Journal of Cell Biology, 163(4), 889-99. [DOI:10.1083/jcb.200307068] [PMID] [PMCID]
- Daly, W., Yao, L., Zeugolis, D., Windebank, A., & Pandit, A. (2012). A biomaterials approach to peripheral nerve regeneration: Bridging the peripheral nerve gap and enhancing functional recovery. Journal of the Royal Society Interface, 9(67), 202-21. [DOI:10.1098/rsif.2011.0438] [PMID] [PMCID]
- de Blaquiere, G. E., Curtis, J., Pereira, J. H., & Turk, J. L. (1994). Denatured muscle grafts for nerve repair in an experimental model of nerve damage in leprosy. 1. A functional and morphometric study. “International Journal of Leprosy and Other Mycobacterial Diseases, 62(1), 55-63. [PMID]
- Delaviz, H., Faghihi, A., Delshad, A. A., hadi Bahadori, M., Mohamadi, J., & Roozbehi, A. (2011). Repair of peripheral nerve defects using a polyvinylidene fluoride channel containing nerve growth factor and collagen gel in adult rats. Cell Journal (Yakhteh), 13(3), 137-42. [PMID] [PMCID]
- Delaviz, H., Joghataie, M. T., Mehdizadeh, M., Bakhtiyari, M., Nobakht, M., & Khoei, S. (2008). Transplantation of olfactory mucosa improve functional recovery and axonal regeneration following sciatic nerve repair in rats. Iranian Biomedical Journal, 12(4), 197-202. [PMID]
- Dodla, M. C., & Bellamkonda, R. V. (2008). Differences between the effect of anisotropic and isotropic laminin and nerve growth factor presenting scaffolds on nerve regeneration across long peripheral nerve gaps. Biomaterials, 29(1), 33-46. [DOI:10.1016/j.biomaterials.2007.08.045] [PMID] [PMCID]
- Glasby, M., Gschmeissner, S., Hitchcock, R., & Huang, C. (1986). Regeneration of the sciatic nerve in rats. The effect of muscle basement membrane. Bone & Joint Journal, 68(5), 829-33. [DOI:10.1302/0301-620X.68B5.3782256]
- Glasby, M., Gschmeissner, S., Huang, C. H., & De Souza, B. (1986). Degenerated muscle grafts used for peripheral nerve repair in primates. Journal of Hand Surgery (European Volume), 11(3), 347-51. [DOI:10.1016/0266-7681(86)90155-5]
- Glasby, M. A., Gschmeissner, S. E., Huang, C. L., & De Souza, B. A. (1986). Degenerated muscle grafts used for peripheral nerve repair in primates. The Journal of Hand Surgery: British & European Volume, 11(3), 347-51. [DOI:10.1016/0266-7681(86)90155-5]
- Glasgow, H. L., Whitney, M. A., Gross, L. A., Friedman, B., Adams, S. R., Crisp, J. L., et al. (2016). Laminin targeting of a peripheral nerve-highlighting peptide enables degenerated nerve visualization. Proceedings of the National Academy of Sciences, 113(45), 12774-9. [DOI:10.1073/pnas.1611642113] [PMID] [PMCID]
- Hedayatpour, A., Sobhani, A., Bayati, V., Abdolvahhabi, M. A., Shokrgozar, M. A., & Barbarestani, M. (2007). A method for isolation and cultivation of adult Schwann cells for nerve conduit. Archives of Iranian Medicine, 10(4), 474-80. [PMID]
- Heermann, S., & Schwab, M. H. (2013). Molecular control of Schwann cell migration along peripheral axons: keep moving! Cell Adhesion & Migration, 7(1), 18-22. [DOI:10.4161/cam.22123] [PMID] [PMCID]
- Houštava, L., Dubový, P., Haninec, P., & Grim, M. (1999). An alternative preparation of the acellular muscle graft for reconstruction of the injured nerve-morphological and morphometric analysis. Annals of Anatomy-Anatomischer Anzeiger, 181(3), 275-81. [DOI:10.1016/S0940-9602(99)80043-0]
- Iijima, Y., Ajiki, T., Murayama, A., & Takeshita, K. (2016). Effect of Artificial Nerve Conduit Vascularization on Peripheral Nerve in a Necrotic Bed. Plastic and Reconstructive Surgery Global Open, 4(3), e665. [DOI:10.1097/GOX.0000000000000652] [PMID] [PMCID]
- Kang, X. W., Hu, J. L., Wang, S. K., & Wang, J. (2015). Effectiveness of muscle basal lamina carrying neural stem cells and olfactory ensheathing cells in spinal cord repair. Genetics and Molecular Research, 14(4), 13437-55. [DOI:10.4238/2015.October.28.5] [PMID]
- La Fleur, M., Underwood, J. L., Rappolee, D. A., & Werb, Z. (1996). Basement membrane and repair of injury to peripheral nerve: defining a potential role for macrophages, matrix metalloproteinases, and tissue inhibitor of metalloproteinases-1. Journal of Experimental Medicine, 184(6), 2311-26. [DOI:10.1084/jem.184.6.2311] [PMID] [PMCID]
- Labrador, R. O., Butı́, M., & Navarro, X. (1998). Influence of collagen and laminin gels concentration on nerve regeneration after resection and tube repair. Experimental Neurology, 149(1), 243-52. [DOI:10.1006/exnr.1997.6650] [PMID]
- Ma, F., Zhu, T., Xu, F., Wang, Z., Zheng, Y., Tang, Q., et al. (2016). Neural stem/progenitor cells on collagen with anchored basic fibroblast growth factor as potential natural nerve conduits for facial nerve regeneration. Acta Biomaterialia, 50, 188-97. [DOI:10.1016/j.actbio.2016.11.064] [PMID]
- Ma, S., Peng, C., Wu, S., Wu, D., & Gao, C. (2013). Sciatic nerve regeneration using a nerve growth factor-containing fibrin glue membrane. Neural Regeneration Research, 8(36), 3416-22. [PMID] [PMCID]
- Madison, R. D., & Robinson, G. A. (2014). Accuracy of regenerating motor neurons: Influence of diffusion in denervated nerve. Neuroscience, 273, 128-40. [DOI:10.1016/j.neuroscience.2014.05.016] [PMID] [PMCID]
- Mafi, P., Hindocha, S., Dhital, M., & Saleh, M. (2012). Suppl 1: Advances of peripheral nerve repair techniques to improve hand function: A systematic review of literature. The Open Orthopaedics Journal, 6, 60-8. [DOI:10.2174/1874325001206010060] [PMID] [PMCID]
- Manthorpe, M., Engvall, E., Ruoslahti, E., Longo, F. M., Davis, G. E., & Varon, S. (1983). Laminin promotes neuritic regeneration from cultured peripheral and central neurons. The Journal of Cell Biology, 97(6), 1882-90. [DOI:10.1083/jcb.97.6.1882] [PMID]
- Meek, M. F. (2000). Effects of nerve growth factor on nerve regeneration through a vein graft across a gap. Plastic and Reconstructive Surgery, 106(5), 1227-8. [DOI:10.1097/00006534-200010000-00065] [PMID]
- Meek, M. F., Varejão, A. S., & Geuna, S. (2004). Use of skeletal muscle tissue in peripheral nerve repair: Review of the literature. Tissue Engineering, 10(7-8), 1027-36. [DOI:10.1089/1076327041887655] [PMID]
- Mesentier-Louro, L. A., De Nicolò, S., Rosso, P., De Vitis, L. A., Castoldi, V., Leocani, L., et al. (2017). Time-dependent nerve growth factor signaling changes in the rat retina during optic nerve crush-induced degeneration of retinal ganglion cells. International Journal of Molecular Sciences, 18(1), 98. [DOI:10.3390/ijms18010098] [PMID] [PMCID]
- Mohammadi, J., Delaviz, H., Mohammadi, B., Delaviz, H., & Rad, P. (2016). Comparison of repair of peripheral nerve transection in predegenerated muscle with and without a vein graft. BMC Neurology, 16, 237. [DOI:10.1186/s12883-016-0768-z] [PMID] [PMCID]
- Norris, R., Glasby, M., Gattuso, J., & Bowden, R. (1988). Peripheral nerve repair in humans using muscle autografts. A new technique. Bone & Joint Journal, 70(4), 530-3. [DOI:10.1302/0301-620X.70B4.3403592]
- Panagopoulos, G. N., Megaloikonomos, P. D., & Mavrogenis, A. F. (2017). The present and future for peripheral nerve regeneration. Orthopedics, 40(1):e141-e56. [DOI:10.3928/01477447-20161019-01] [PMID]
- Pereira, J. H., Bowden, R. E., Narayanakumar, T. S., & Gschmeissner, S. E. (1996). Peripheral nerve reconstruction using denatured muscle autografts for restoring protective sensation in hands and feet of leprosy patients. Indian Journal of Leprosy, 68(1), 83-91. [PMID]
- Perretta, D., & Green, S. (2017). Bridging the gap in peripheral nerve repair. Bulletin of the NYU Hospital for Joint Diseases, 75(1), 57-63. [PMID]
- Roganovic, Z., Ilic, S., & Savic, M. (2007). Radial nerve repair using an autologous denatured muscle graft: Comparison with outcomes of nerve graft repair. Acta Neurochirurgica, 149(10), 1033-9. [DOI:10.1007/s00701-007-1269-z] [PMID]
- Roozbehi, A., Joghataie, M. T., Mehdizadeh, M., Mirzaei, A., & Delaviz, H. (2012). The effects of cyclosporin-A on functional outcome and axonal regrowth following spinal cord injury in adult rats. Acta Medica Iranica, 50(4), 226-32. [PMID]
- Sadraie, S. H., Parivar, K., Arabi, F., Moattari, M., Kaka, G., & Mansouri, K. (2016). Study of transected sciatic nerve repair by amniotic membrane with betamethasone in adult albino wistar rats. Archives of Iranian Medicine, 19(9), 612-7. [DOI:0161909/AIM.003] [PMID]
- Sanes, J. R. (2003). The basement membrane/basal lamina of skeletal muscle. Journal of Biological Chemistry, 278(15), 12601-4. [DOI:10.1074/jbc.R200027200] [PMID]
- Sullivan, R., Dailey, T., Duncan, K., Abel, N., & Borlongan, C. (2016). Peripheral nerve injury: Stem cell therapy and peripheral nerve transfer. International Journal of Molecular Sciences, 17(12), 2101. [DOI:10.3390/ijms17122101] [PMID] [PMCID]
- Takami, T., Oudega, M., Bates, M. L., Wood, P. M., Kleitman, N., & Bunge, M. B. (2002). Schwann cell but not olfactory ensheathing glia transplants improve hindlimb locomotor performance in the moderately contused adult rat thoracic spinal cord. Journal of Neuroscience, 22(15), 6670-81. [DOI:10.1523/JNEUROSCI.22-15-06670.2002] [PMID]
- Turgut, M., & Kaplan, S. (2011). Effects of melatonin on peripheral nerve regeneration. Recent Patents on Endocrine, Metabolic & Immune Drug Discovery, 5(2), 100-8. [DOI:10.2174/187221411799015336] [PMID]
- Valentini, R., Aebischer, P., Winn, S., & Galletti, P. (1987). Collagen-and laminin-containing gels impede peripheral nerve regeneration through semipermeable nerve guidance channels. Experimental Neurology, 98(2), 350-6. [DOI:10.1016/0014-4886(87)90247-0]
- Verdú, E., Labrador, R. O., Rodríguez, F. J., Ceballos, D., Forés, J., & Navarro, X. (2002). Alignment of collagen and laminin-containing gels improve nerve regeneration within silicone tubes. Restorative Neurology and Neuroscience, 20(5), 169-80. [PMID]
- Wallquist, W., Patarroyo, M., Thams, S., Carlstedt, T., Stark, B., Cullheim, S., et al. (2002). Laminin chains in rat and human peripheral nerve: Distribution and regulation during development and after axonal injury. Journal of Comparative Neurology, 454(3), 284-93. [DOI:10.1002/cne.10434] [PMID]
- Yang, X. N., Jin, Y. Q., Bi, H., Wei, W., Cheng, J., Liu, Z. Y., et al. (2013). Peripheral nerve repair with epimysium conduit. Biomaterials, 34(22), 5606-16. [DOI:10.1016/j.biomaterials.2013.04.018] [PMID]
- Zarinfard, G., Tadjalli, M., Razavi, S., & Kazemi, M. (2016). Effect of laminin on neurotrophic factors expression in schwann-like cells induced from human adipose-derived stem cells in vitro. Journal of Molecular Neuroscience, 60(4), 465-73. [DOI:10.1007/s12031-016-0808-6] [PMID]