1. Introduction
Unlike acquiring the first Language (L1), which is commonly considered natural and effortless, adult second Language (L2) learning is relatively laborious. Some scholars believe that adults cannot learn a second language using the same neurocognitive mechanisms children rely on for their first language (
Lenneberg, 1967). According to
Paradis (1994,
2004), L1 is generally acquired implicitly. In contrast, a second language will be acquired explicitly through different brain mechanisms if learned after a critical period (around the age of puberty). However, recent evidence indicates that even for language aspects such as grammar, L1-like brain processing may eventually be attained (Gilon-Dowens, Vergara, Barber, & Carreiras, 2010;
Hahne, Mueller, & Clahsen, 2006;
Steinhauer, White, & Drury, 2009).
So far, many Second Language Acquisition (SLA) theories have accounted for the L1 versus L2 learning differences. For example, the proponents of the Critical Period Hypothesis (CPH) address the presence of a window of opportunity showing a limited developmental sensitive period beyond which the ability to learn language declines and consequently the ultimate L1 native-like proficiency seems far-fetched for L2 learners (
Lenneberg, 1967). On the other hand, the Declarative/Procedural (DP) model (
Ullman, 2001) proposes that L1 and L2 are initially processed by different underlying systems. While syntactic processing in L1 is procedural, L2 processing relies on declarative memory in the initial stages. However, with increasing proficiency in L2, syntactic processes shift to the more procedural memory system as individuals begin learning rules rather than memorizing words and phrases. Declarative Memory (DM) system includes semantic knowledge (knowledge about facts) and episodic knowledge (knowledge about events) and is responsible for very rapid learning (Ullman, 2004). At least part of this knowledge can be consciously and explicitly recollected. The medial temporal lobe structures are where DM is located, i.e. the hippocampal region, entorhinal cortex, perirhinal cortex, parahippocampal gyrus, and anterior/ventral inferior frontal cortex (BA 45/47) (
Ullman, 2001). Procedural Memory (PM) comprises a network of brain structures rooted in frontal/basal-ganglia circuits, with a potential role for portions of the parietal cortex, superior temporal cortex, and the cerebellum. PM is related to the Broca’s Area (BA 44)) (
Rizzolatti, Fogassi, & Gallese, 2000; (
Ullman, 2004). PM is assumed to play a role in all sub-domains of grammar, which depends on several functions: syntax (inflectional and derivational morphology), regular forms (
Ullman, 2001) as well as irregulars that appear to be affixed, aspects of phonology (the combination of sounds), and possibly non-lexical semantics (aspects of the composition of words into complex structures).
Several bilingual language investigations have reported differences in the pattern of cerebral activation associated with the bilingual subjects’ L1 versus L2 using various neuroimaging methods (
Perani et al., 1998). The different activation patterns could be ascribed to several confounding conditions, e.g. the Age of Acquisition (AoA), proficiency level, type of instruction (implicit vs. explicit), and so on. These studies show that L2 proficiency modulates the Event-Related Potential (ERP) signatures associated with L2 syntactic processing. In this regard, high proficient L2 learners often display L1-like patterns, whereas the ERP responses of low-proficient L2 learners demonstrate quantitative and qualitative differences (
Diaz et al., 2016). Additionally, comparing with semantic processing, ERP effects of syntactic processing are more affected by AoA (
Hahne & Friederici, 2001).
More significant differences between L1 and L2 in syntactic processing, but not semantic processing, suggest that while the same underlying structures (i.e. the declarative memory system) are responsible for semantic processing, L1 and L2 syntactic processing rely on different structures. That is, while syntactic processing in L1 is procedural, it relies on declarative memory in L2. The DP model describes both syntax and semantics and can account for the effect of individual differences on bilingual language processing. Similarly, some other SLA theories emphasize the distinction between these two memory systems, e.g. De Keyser’s (2007) skill acquisition theory,
Anderson’s (1993,
2013) ACT (adaptive control of thought) model,
Ellis’ (2008) model of implicit and explicit knowledge, and
Paradis (1994,
2004,
2009,
2013). Based on these theories, the two systems play a crucial role in language learning in a way that early L2 development is dependent on declarative memory, while later stages of learning are more dependent on procedural memory as the individual gains more proficiency in L2. As a consequence of increased exposure, experience, and proficiency, L2 learners rely more on their procedural (non-declarative) and implicit knowledge. Another confounding condition accounting for L1 vs. L2 learning differences is the learning context. For example, studies have shown that under implicit training conditions, L2 acquisition at later stages involves procedural memory functioning. However, when adult learners are exposed to a second language under explicit instruction of grammatical rules in the classroom context, the evidence suggests different L2 learning and neurocognitive outcomes from L1 (
Brill-Schuetz & Morgan-Short, 2014).
ERP research has provided us with several activation patterns as a clear reference for examining the attainment of native language processing in L2 studies (
Friederici, Steinhauer, & Pfeifer, 2002). Difficulties in lexical/semantic processing in L1 elicit central or posterior bilaterally-distributed negativities (N400). Fkjfhgor semantic violations, in both L1 and L2, N400 was elicited even after minimal L2 exposure, although in some cases, the N400 in L2 was delayed or last longer (
Steinhauer et al., 2009;
Ullman, 2001). On the contrary, L2 differs from L1 in syntactic processing aspects, particularly at lower levels of proficiency in which Anterior Negativities (ANs) do not appear. Instead, participants show N400s or N400-like posterior negativities and a typically larger positivity for higher proficient bilinguals in response to syntactic violations (P600) (
Osterhout et al., 2008). P600 is associated with the activation of procedural memory areas while processing violated L2 regular past tense and phrase structures, although inconsistent results have been reported about this component (Ullman, 2003,
2006, 2015). Accordingly, this study was conducted to investigate the significant differences between the ERP components elicited from the processing of English regular past tense verbs, phrase structure, and final semantic word violations in pre-intermediate and high proficient adult L2 learners.
2. Methods
2.1. Participants
A total number of 20 healthy right-handed adult subjects (L1=Persian, L2=English; 10 high-proficient/Advanced (Ad), 10 Pre-Intermediate (PI) levels; the age of learning onset >15 years; Gender = Female; mean age=25.50 years, SD = 5.09 years, age range = 19-35 years of age), without any neurological or psychiatric pathology, were enrolled in this study. Sampling was non-probability purposive. The initial population included 51 Persian EFL (English as a foreign language) learners who filled out the Language Experience and Proficiency Questionnaire (LEAP-Q). For assessing language profiles and demographic questions in bilinguals and multilingual (
Marian, Blumenfeld, & Kaushanskaya, 2007), they did the Edinburgh inventory of handedness (
Oldfield, 1971) and took the online Oxford Placement Test (OPT).
The subjects were assigned into two groups of Pre-Intermediate (B1) and high proficient (C1) levels based on the Common European Framework of reference (CEFR) (N=14 in each group). Eight subjects were selected randomly (four from each group) for piloting the task conditions in terms of both behavioral data and the ERPs. Extracted data from these subjects were not included in the final study analysis due to the learning and noticing effect. Some variables were controlled through the demographic questionnaire. The age of acquisition, i.e. the age of onset for participants in both groups, was over 15 years (around puberty). The pre-intermediate group had the language experience of fewer than 5 years, and the advanced group had the experience of learning English of between 5 to 8 years. The educational level of all participants was either a BA or MA in Psychology, Linguistics, Engineering, Neuroscience, and Law. They all learned English explicitly through English language institutes or school courses and mainly through the grammar-translation methods, therefore, regarded as “compound bilinguals” (
Nilipour, 1973, p. 3). They all knew only two languages, and their mother tongue was Persian. They either volunteered or accepted to participate for monetary rewards. All participants signed a standard international consent based on the Declaration of Helsinki for Medical Studies (2018).
2.2. Stimuli
The ERP task data (including the list of sentences for all 6 conditions) were adopted from
Newman, Tremblay, Nichols, Neville, and Ullman (2012). The list consisted of 240 simple declarative English sentences, 40 in each of the 6 conditions (
Table 1).
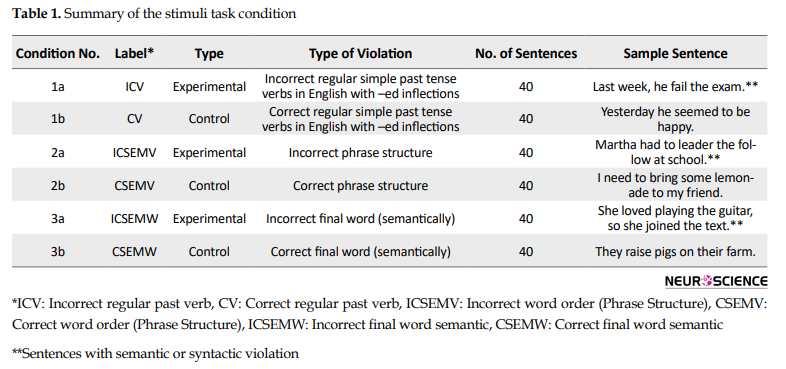
For each correct sentence, an anomalous version was created to correspond to the experimental version. Stimuli were counterbalanced across subjects such that each subject saw only the control or the anomalous version of a given sentence. Condition 1b (CV) included 40 sentences (control) with the regular past tense verb; all sentences had similar structures, beginning with “Yesterday…” or “Last week…” or “any other past tense marker adverb,” followed by a pronoun (I, he, she, etc.), the verb, and then the post-verbal argument. For each sentence, a corresponding anomalous experimental sentence was created by replacing the past tense inflected form of the verb with its stem (unmarked) form (e.g. Last week, he fail the exam.) (Condition 1a, ICV). In condition 2a (ICSEMV), 40 sentences (experimental) belonged to the syntactic condition in which phrase structure anomalies were created by reversing the order of the object noun and the closed-class function word following it, e.g. “Yesterday I cut Max’s with apple caution” instead of apple with”. Or “Martha had to leader the follow at school” instead of follow the leader. Condition 2b (CSEMV) included 40 sentences of the similar phrase structure as condition 2b, only with correct sentences. Condition 3a (ICSEMW) included 40 experimental sentences with the anomalous version of each being created by replacing the object noun in the final position of the sentence with another noun that was semantically incongruent, given the preceding context, e.g. “Yesterday I sailed Todd’s hotel instead of boat”. All sentences in conditions 1a and 1b, and 3a and 3b had similar structures, consisting of two words (including the grammatical subject), followed by a verb and a proper noun (except in the case of phrase structure violations of conditions 2a and 2b, where the violation was produced by swapping the positions of this noun and the following closed-class word). Correctly-formed control sentences for the phrase structure and lexical-semantic conditions were quite similar to each other.
The presentation of each sentence was initiated by the subject’s pressing either of the two response buttons, which caused the outline of a box (7×3 degrees of visual angle) to appear in the center of the screen, followed by the first word after a variable (random) delay of 300-1100 ms to attenuate ERP effects associated with the expectation of forthcoming stimuli. Each trial (word) was shown to the participants at a rate found to be comfortable for them in the pilot testing of the task by
Newman et al. (2012). The trial presentation duration was 300 ms (SOA 500 ms). To forestall subjects’ responding, and possibly generating ERP artifacts, the box outline remained on the screen for a random period of 300 to1100 ms following the onset of the final word of each sentence. The words of the sentence were presented one at a time, with each word displayed for 300 ms and a 200-ms delay between words. The outline of the box remained for 1500 ms after the last word of the sentence. After the box disappeared from the screen, the correct or incorrect sentence should be pointed via a right click or left click, respectively. After the last word of each sentence, the fixation cross remained on the screen for an additional 500 ms. Afterward, the participants had up to 5 seconds to make a judgment about whether the sentence was correct or incorrect, using the buttons of a computer mouse. The next sentence and the fixation cross came up immediately after the response.
2.3. The ERP recording
The recording duration was 25-30 minutes using an EEG amplifier (Mitsar) and the WinEEG software at the Psychology Lab of Shahid Beheshti University, Department of Psychology, Tehran, Iran. The stimuli were presented visually on an 18.5” computer screen of 1369×768 Pixels quality using Psytask 1.52. The stimuli were given, and data were recorded via a dual-core Pentium computer under Windows 7 Ultimate.
The EEG cap of the right size was placed on the subjects’ head and then attached to the EEG electrodes while the subjects were sitting still on chairs. The subjects were asked to fixate their eye movement into the screen and push the right and the left button on the computer mouse without moving their hands, body, or eyes. The right bottom entailed the correctness of the trials, and the left bottom represented the incorrect responses. There was a pilot of random 10 sentences for each subject to warm up and practice the procedure. The subjects had two intervals when the researcher paused the recording and gave them drinks or food to prevent fatigue or muscle tension. After the data were recorded, they were extracted in EDF1 format and provided for the data analysis.
Continuous ERP data were recorded from each participant via 32 tin electrodes, only 19 of which were active. Electrode positions were specified by the international 10–20 system (FP1, FP2, FPz, Fz, F3, F4, FCz, C3, Cz, C4, CPz, P3, T3, T4, T5, T6, Pz, P4, O1, O2 left/right auricles). Impedances were lowered to <5 kΩ. EEG was amplified using an online bandpass filter of 208 Hz. Trials with blinks, eye movements, or excessive noise were identified off-line (using a maximum peak-to-peak amplitude threshold tailored to each participant’s data) and discarded.
2.4. Data analysis
A 2-sample t test was used to compare the ERP latency and amplitude of the two groups on each condition, and each group’s performance on the violated and correct sets of conditions. One-way ANOVA was used to compare the ERPs of the groups across different conditions. ERPs to target words in each condition were elicited across the N400 time window (300-500 ms) and the P600 time window (500-700 ms), i.e. 100 ms before and after the target word.
3. Results
3.1. Between-group comparisons
Figures 1 and 2 show the distribution of t value for the comparison of P600 (peak latency and amplitude, respectively) between the Ad and PI subjects in all 6 conditions. In general, the results showed that the P600 latency between Ad and PI subjects was different for the conditionsshown in
Table 2 among the experimental conditions, the t value for P600 peak latency was different significantly only for the incorrect past tense verb (ICV) condition and in O2 (P=0.039463, t=2.2205, confidence interval: 0.003112 - 0.11249, P<0.05) between the two groups (higher in the advanced group).
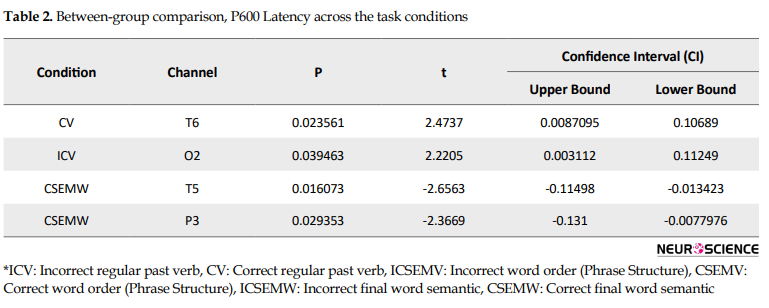
For correct sentences in all conditions, the P600 peak amplitude was smaller for the Ad group as compared with the PI (
Table 3).
P600 peak amplitude between the Ad and PI subjects was significantly different for the correct final semantic word sentences (CSEMW) and correct phrase structure (CSEMV) conditions (P<0.05).
Results (
Table 4) showed that the t-value for N400 peak latency differed between the two groups (higher in the advanced group) in correct and incorrect past tense verb (CV & ICV), correct and incorrect phrase structure (CSEMV & ICSEMV), and Incorrect Semantic Final Word (ICSEMW) conditions.
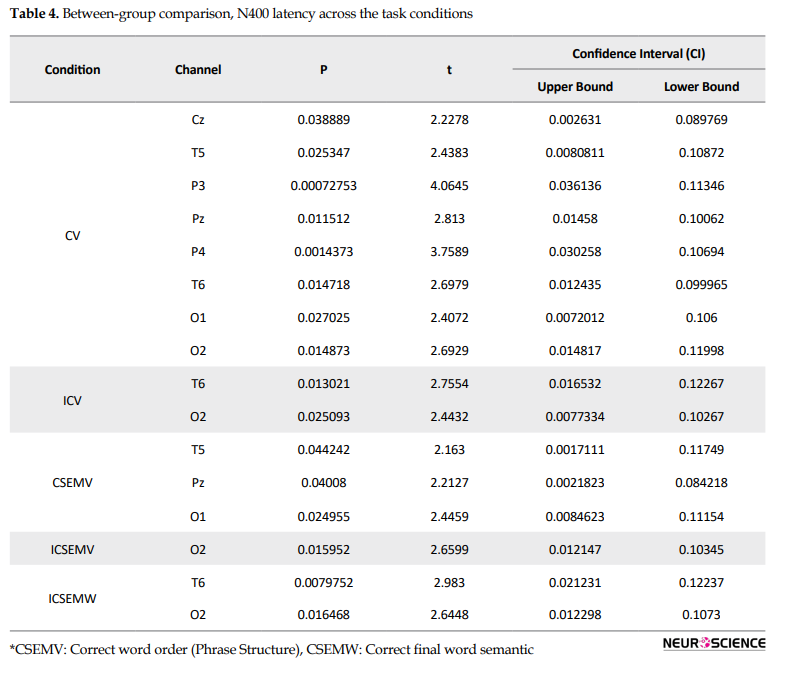
In all of the experimental conditions significant difference was seen in T6 and O2 regions, i.e. for ICV (T6, Pval=0.013021, tval=2.7554, confidence interval: 0.016532-0.12267; and O2, Pval=0.025093, tval=2.4432, confidence interval: 0.0077334- 0.10267, P <0.05), for ICSEMV (O2, Pval=0.015952, tval=2.6599, confidence interval: 0.012147-0.10345, P <0.05), and for ICSEMW (T6, Pval=0.0079752, tval=2.983, confidence interval: 0.021231-0.12237; and O2, Pval=0.016468, tval=2.6448, confidence interval: 0.012298-0.1073, P <0.05).
N400 latency between Ad and PI subjects was different in T5, Pz, and O1 for condition 3b (CSEMW). Results showed that the incorrect semantic sentences increased the latency of N400 in the Ad group. More significant differences were found for the correct sentences than the incorrect ones. Semantic processing caused some significant differences in the temporal, parietal, and occipital regions. T5, Pz, and O1 for correct sentences and O2 (ICSEMW, O2: Pval=0.015952, tval=2.6599, confidence interval: 0.012147 - 0.10345) for incorrect sentences showed significant differences in N400 latency between the Ad and PI groups (
Table 3).
Figure 3 shows the t value distribution when the N400 latency was compared between the Ad and PI groups.
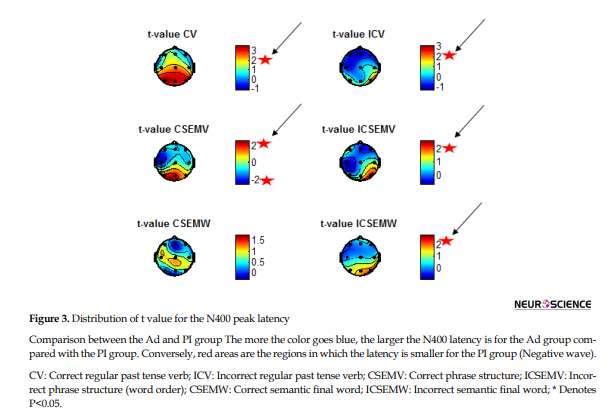
The N400 peak amplitude between the Ad and PI subjects was significantly different for all control and experimental conditions (
Table 5).
Besides, as can be seen in
Figure 4, the N400 amplitude in the frontal region was larger in the Ad compared to that in the PI group.
The more the color goes blue, the larger the N400 amplitude is for the Ad group compared with the PI group. Conversely, red areas are the regions where peak amplitude is smaller for the PI group (Negative wave).
3.2. Within-Group Comparisons
Figure 5 shows the difference between the ERP data elicited by the experimental and control conditions related to the processing of regular English past tense verbs (ICV & CV) by the Ad subjects.
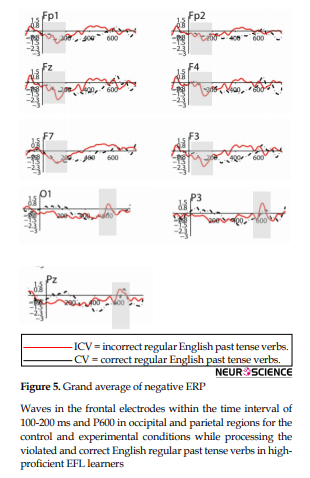
Within the time window of 100-200 ms, a negative component appeared in the frontal regions (Fp1, Fp2, Fp3, F7, F4, & Fz) upon processing the incorrect English regular past tense verbs in high-proficient EFL learners. Also, the grand average results for the Ad group show P600 in Pz, P3, and O1 during the processing of the incorrect regular past verb.
Figure 6 shows the difference between the N400 amplitude elicited by the experimental and control conditions related to the processing of correct and incorrect phrase structure by the Ad subjects in P3 and T5 electrodes; however, further statistical analysis showed that this difference was only statistically significant in T5.
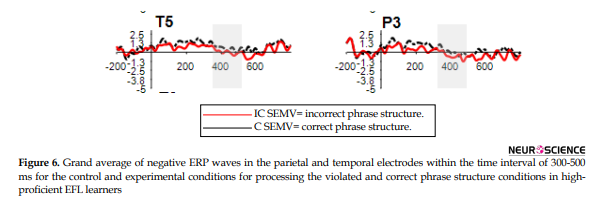
Within the time window of 100-200 ms, a negative component (N400) appeared in the frontal regions (FZ, F4, F7, FP1, FP2, F3) upon the processing of Incorrect Final Word Semantic Sentences (ICSEMW) in the Ad subjects. However, no statistically significant N400 difference was observed in the Ad subjects while processing correct and incorrect final word semantic sentences. On the other hand, only at T3, there was a significant P600 amplitude difference between the two conditions (CSEMW & ICSEMW) (
Figure 7).
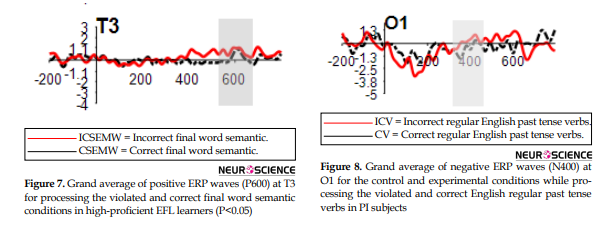
Figure 8 shows the significant difference in N400 amplitude for the incorrect regular past conditions only in O1 for the PI subjects.
P600 amplitude did not differ significantly between the two conditions for these subjects; however, not significantly, the P600 latency in F7 was bigger for the PI as compared with the Ad subjects.
N400 amplitude for PI subjects was different between CSEMV and ICSEMV conditions in O2 (Pval: 0.034792, tval: 2.4835, confidence interval: 0.069237-1.4846, P <0.05); P600 amplitude for the PI subjects was different between CSEMV and ICSEMV conditions in T4 (Pval: 0.024103, tval: 2.7073, confidence interval: 0.16136-1.8012, P <0.05) (
Figure 9).
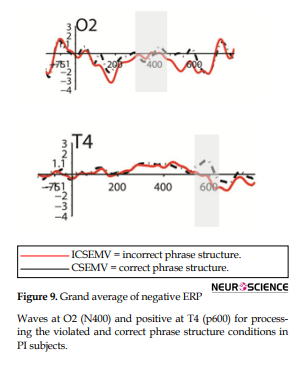
There was no significant P600 and N400 amplitude difference between the CSEMW and ICSEMW conditions for the PI subjects.
4. Discussion
A negative component appeared in the frontal regions (Fp1, Fp2, Fp3, F7, F4, and Fz) upon processing the incorrect English regular past tense verbs in high-proficient EFL learners, which confirms
Newman, Pancheva, Ozawa, Neville, and Ullman (2001)’s findings in which syntactic violations elicited greater activation than semantic anomalies in several regions of the superior frontal gyrus, in both hemispheres. Besides, the grand average results of our study showed a significant difference in N400 amplitude for the incorrect regular past conditions only in O1 for the PI subjects. This finding is compatible with
Osterhout et al. (2008)’s explanation in that L2 differs from L1 in aspects of syntactic processing, in particular at lower levels of proficiency in which Anterior Negativities (ANs) do not appear and instead participants show N400s or N400-like posterior negativities. This result shows the initial reliance on the declarative memory system for syntactic processing at lower levels of L2. On the contrary, in higher L2 proficiency levels, ANs are reported (Gilon-Dowens et al., 2010), which shows the reliance on native language processing mechanisms in syntactic processing (i.e. procedural memory and the basal ganglia) for more proficient L2 users.
In addition, the grand average results showed P600 amplitude differences in Pz, P3, and O1 during the processing of the incorrect regular past verb for the Ad subjects of this study. And it did not differ significantly between the correct and incorrect regular past tense conditions of the PI group. Instead, during the processing of the incorrect regular past verbs, the P600 latency in F7 was bigger for the PI as compared with the Ad subjects, which could reflect the time the PI subjects needed for retrieval of the elements. This larger P600 latency for the PI subjects could be explained in light of the discussions of the P600 by
Friederici et al. (2002) and
Hagoort (2003). They claimed that if the P600 reflects the creation or destruction of syntactic relations, then the latency of the P600 should reflect the time needed for retrieval of the elements that participate in those relations. In contrast, the duration and amplitude of the P600 should be a function of the structure-building processes themselves. They then predicted that different structural and lexical manipulations should impact the P600 differently. Manipulations that impact retrieval processes should change the latency of the P600. In contrast, manipulations that impact the number and type of syntactic relations should change the amplitude and or duration of the P600.
Our findings showed that within the time window of 300-500 ms, a negative component appeared in the parietal regions (P3, Pz, and P4) upon the processing of both correct and incorrect phrase structures. The negative wave was stronger in the case of correct sentences as opposed to the incorrect word orders, which contrasts with
Weber-Fox and Neville (1996)’s who reported no early negativity compared to monolinguals in all but one of the 5 groups of L2 learners in the phrase structure condition. This part of our findings is not consistent with the critical period hypothesis, which maintains that there is a biologically determined critical period in language acquisition, implying that late learners of an L2 cannot achieve native-like proficiency. Our findings also showed that word order violation caused another negative component, mostly in the frontal and central regions; furthermore, within the time window of 100-300 ms, a negative component appeared in the left anterior electrodes of F3, T3, FP1, and F7. This finding is consistent with
Newman, Pancheva, Waligura, and Neville (2001)’s who reported that syntactic violations typically elicit one or more ERP components that are distinct from the N400. The most widely-reported components are left anterior negativities (LANs2-150–500 ms) and later positivities (500-1000 ms), frequently labeled P600s3. A biphasic LAN-late positivity pattern has been observed for phrase structure violations, syntactic agreement violations, and violations of morphological structure. According to one view, early (100-200 ms) LANs, which are frequently observed in response to phrase structure violations, reflect early word category identification and syntactic structure building, while LANs in the 300–500 ms range reflect morphological processing, as well as syntactic and lexical integration (
Friederici, 2002). Left anterior negativities have been observed in correlation with phrase structure violations also in
Friederici, Pfeifer, and Hahne (1993),
Friederici, Hahne, and Mecklinger (1996), and
Münte, Heinze, and Mangun (1993).
Moreover, P600 latency showed no significant differences between the two groups of subjects during the final word semantic condition. P600 peak amplitude between the Ad and PI subjects was significantly different for the correct final semantic word sentences only (F4 and Pz). This finding agrees with
Newman et al. (2007). They asserted that since the P600 was first identified, it has been widely assumed to be observed in anomalous or difficult sentences that reflect syntactic processes.
Our analysis showed that the latency of the P600 component for incorrect regular past tense sentences in the occipital region (O2) was significantly higher in the Ad than in the PI group. Besides, based on the t value results and the confidence intervals obtained, it can be deduced that the P600 amplitude was smaller for the Ad group than the PI group when the correct sentences were shown to subjects. In other words, it seems that the PI subjects did expect to observe a conceptual error (semantic violation) because they did not have sufficient English control in correct sentences. However, the statistical analysis does not show a significant difference in the P600 amplitude between the Ad and PI groups while processing sentences with violations. It is important to note that this finding does not mean the absence of the P600 component. Rather it implies that the amplitude of this component between the two groups was not significantly different.
Ethical Considerations
Compliance with ethical guidelines
All ethical principles are considered in this article. The participants were informed about the purpose of the research and its implementation stages.
Funding
This research did not receive any grant from funding agencies in the public, commercial, or non-profit sectors.
Authors' contributions
Conceptualization and methodology: Reza Nilipour; Investigation and Validation: Laleh Esfandiari, Reza Nilipour, Reza Khosrowabadi, and Vahid Nejati; Writing the original draft, review, and editing: Laleh Esfandiari; Statistical analysis and interpretation: Reza Khosrowabadi; Supervision and Visualization: All Authors.
Conflict of interest
The authors declared no conflict of interest.
Acknowledgments
Special thanks to dr. Mahdiyeh Esmaili from Shahid Beheshti University for her assistance in designing the ERP task.
References
Anderson, J. R. (1993). Rules of the mind. Mahwah, NJ: Lawrence Erlbaum Associates Inc. https://books.google.com/books?id=1KOYAgAAQBAJ&printsec=frontcover&dq
Anderson, J. R. (2013). The architecture of cognition. New York: Psychology Press. [DOI:10.4324/9781315799438]
Brill-Schuetz, K., & Morgan-Short, K. (2014). The role of procedural memory in adult second language acquisition. In. Proceedings of the Annual Meeting of the Cognitive Science Society, 36(36), 1-7. https://escholarship.org/content/qt0dc7958r/qt0dc7958r.pdf
DeKeyser, R. (2007). Practice in a second language: Perspectives from applied linguistics and cognitive psychology. Cambridge: Cambridge University Press. [DOI:10.1017/CBO9780511667275]
Díaz, B., Erdocia, K., de Menezes, R. F., Mueller, J. L., Sebastián-Gallés, N., & Laka, I. (2016). Electrophysiological correlates of second-language syntactic processes are related to native and second language distance regardless of age of acquisition. Frontiers in Psychology, 7, 133. [DOI:10.3389/fpsyg.2016.00133] [PMID] [PMCID]
Ellis, N. C. (2008). Implicit and explicit knowledge about language. Encyclopedia of language and education, 6, 1-13. https://moodle.ph-ooe.at/pluginfile.php/114023/mod_resource/content/1/Ellis_language_acquisition_grammar.pdf
Friederici, A. D. (2002). Towards a neural basis of auditory sentence processing. Trends in Cognitive Sciences, 6(2), 78-84. [DOI:10.1016/S1364-6613(00)01839-8]
Friederici, A. D., Hahne, A., & Mecklinger, A. (1996). Temporal structure of syntactic parsing: Early and late event-related brain potential effects. Journal of Experimental Psychology: Learning, Memory, and Cognition, 22(5), 1219-48. [DOI:10.1037/0278-7393.22.5.1219]
Friederici, A. D., Pfeifer, E., & Hahne, A. (1993). Event-related brain potentials during natural speech processing: Effects of semantic, morphological and syntactic violations. Cognitive Brain Research, 1(3), 183-92. [DOI:10.1016/0926-6410(93)90026-2]
Friederici, A. D., Steinhauer, K., & Pfeifer, E. (2002). Brain signatures of artificial language processing: Evidence challenging the critical period hypothesis. Proceedings of the National Academy of Sciences of the United States of America, 99(1), 529-34. [DOI:10.1073/pnas.012611199] [PMID] [PMCID]
Hagoort, P. (2003). How the brain solves the binding problem for language: A neurocomputational model of syntactic processing. NeuroImage, 20(Suppl 1), S18-29. [DOI:10.1016/j.neuroimage.2003.09.013] [PMID]
Hahne, A., & Friederici, A. D. (2001). Processing a second language: Late learners’ comprehension mechanisms as revealed by event-related brain potentials. Bilingualism: Language and Cognition, 4(2), 123-41. [DOI:10.1017/S1366728901000232]
Hahne, A., Mueller, J. L., & Clahsen, H. (2006). Morphological processing in a second language: Behavioral and event-related brain potential evidence for storage and decomposition. Journal of Cognitive Neuroscience, 18(1), 121-34. [DOI:10.1162/089892906775250067] [PMID]
Lenneberg, E. H. (1967). The biological foundations of language. Hospital Practice, 2(12), 59-67. [DOI:10.1080/21548331.1967.11707799]
Marian, V., Blumenfeld, H. K., & Kaushanskaya, M. (2007). The Language Experience and Proficiency Questionnaire (LEAP-Q): Assessing language profiles in bilinguals and multilinguals. Journal of Speech, Language, and Hearing Research, 50(4), 940-67. [DOI:10.1044/1092-4388(2007/067)]
Münte, T. F., Heinze, H. J., & Mangun, G. R. (1993). Dissociation of brain activity related to syntactic and semantic aspects of language. Journal of Cognitive Neuroscience, 5(3), 335-44. [DOI:10.1162/jocn.1993.5.3.335] [PMID]
Newman, A. J., Pancheva, R., Ozawa, K., Neville, H. J., & Ullman, M. T. (2001). An event-related fMRI study of syntactic and semantic violations. Journal of Psycholinguistic Research, 30(3), 339-64. [DOI:10.1023/A:1010499119393] [PMID]
Newman, A. J., Tremblay, A., Nichols, E. S., Neville, H. J., & Ullman, M. T. (2012). The influence of language proficiency on lexical semantic processing in native and late learners of English. Journal of Cognitive Neuroscience, 24(5), 1205-23. [DOI:10.1162/jocn_a_00143] [PMID] [PMCID]
Newman, A. J., Ullman, M. T., Pancheva, R., Waligura, D. L., & Neville, H. J. (2007). An ERP study of regular and irregular English past tense inflection. NeuroImage, 34(1), 435-45. [DOI:10.1016/j.neuroimage.2006.09.007] [PMID] [PMCID]
Nilipour, R. (1973). Second language teaching method and linguistic interference. Jundi Shapur Educational Journal, 1(1), 1-17.
Oldfield, R. C. (1971). The assessment and analysis of handedness: The Edinburgh inventory. Neuropsychologia, 9(1), 97-113. [DOI:10.1016/0028-3932(71)90067-4]
Osterhout, L., Poliakov, A., Inouea, K., McLaughlina, J., Valentinea, G., & Pitkanena, I., et al. (2008). Second-language learning and changes in the brain. Journal of Neurolinguistics, 21(6), 509-21. [DOI:10.1016/j.jneuroling.2008.01.001] [PMID] [PMCID]
Paradis, M. (1994). Neurolinguistic aspects of implicit and explicit memory: Implications for bilingualism and SLA. In N. C. Ellis (Ed.), Implicit and explicit learning of languages (pp. 393- 420). London: Academic Press. https://books.google.com/books?id=ffHtAAAAMAAJ&dq
Paradis, M. (2004). A neurolinguistic theory of bilingualism. Amsterdam: John Benjamins Publishing Company. [DOI:10.1075/sibil.18]
Paradis, M. (2009). Declarative and procedural determinants of second languages. Amsterdam: John Benjamins Publishing Company. [DOI:10.1075/sibil.40]
Paradis, M. (2013). Late-L2 increased reliance on L1 neurocognitive substrates: A comment on Babcock, Stowe, Maloof, Brovetto & Ullman (2012). Bilingualism: Language and Cognition, 16(3), 704-7. [DOI:10.1017/S1366728913000011]
Perani, D., Paulesu, E., Galles, N. S., Dupoux, E., Dehaene, S., & Bettinardi, V., et al. (1998). The bilingual brain. Proficiency and age of acquisition of the second language. Brain, 121(10), 1841-52. [DOI:10.1093/brain/121.10.1841] [PMID]
Rizzolatti, G., Fogassi, L., & Gallese, V. (2000). Cortical mechanisms subserving object grasping and action recognition: A new view on the cortical motor functions. In M. S. Gazzaniga. The new cognitive neurosciences (pp. 539-551). 2nd Ed. Cambridge, MA: MIT Press. https://books.google.com/books?id=0wx17lC075EC&vq
Steinhauer, K., White, E. J., & Drury, J. E. (2009). Temporal dynamics of late second language acquisition: Evidence from event-related brain potentials. Second Language Research, 25(1), 13-41. [DOI:10.1177/0267658308098995]
Ullman, M. T. (2001). The declarative/procedural model of lexicon and grammar. Journal of Psycholinguistic Research, 30(1), 37-69. [DOI:10.1023/A:1005204207369] [PMID]
Ullman, M. T. (2004). Contributions of memory circuits to language: The declarative/procedural model. Cognition, 92(1-2), 231-70. [DOI:10.1016/j.cognition.2003.10.008] [PMID]
Ullman, M. T. (2006). Is Broca’s area part of a basal ganglia thalamocortical circuit? Cortex, 42(4), 480-5. [DOI:10.1016/S0010-9452(08)70382-4]
Weber-Fox, C. M., & Neville, H. J. (1996). Maturational constraints on functional specializations for language processing: ERP and behavioral evidence in bilingual speakers. Journal of Cognitive Neuroscience, 8(3), 231-56. [DOI:10.1162/jocn.1996.8.3.231] [PMID]