1. Introduction
Wheel Running (WR) and Treadmill Running (TR) are two of the widely-used chronic exercise paradigms in rodent models. TR is considered as a form of forced exercise with fixed parameters such as intensity, duration, and so on while WR is a form of voluntary exercise with less-characterization of parameters in exercise performance (Lin et al., 2012). WR gives the animal the freedom to choose the dosage and the timing of the exercise. On the other hand, forced exercise eliminates the freedom aspect and is usually contingent on an aversive stimulus for behavioral conditioning. In a treadmill, this aversive stimulus is electrical shock (Landers, Kinney, Allen, & van Breukelen, 2013). While the aversive stimulus is known to be effective at conditioning running behavior, it can also lead to stress in the animal (Landers et al., 2013). Voluntary running is done when the animal decides, which normally takes place in the dark cycle whereas, forced running usually happens during the light cycle for convenience (Landers et al., 2013). Rodents are nocturnal animals and less activity is observed during the light cycle and so it is plausible that this may add to the stress they already experience in the forced running (Landers et al., 2013).
Studies with both exercise paradigms have exhibited different effects on anxiety-like behaviors (Burghardt, Fulk, Hand, & Wilson, 2004; Dishman, 1997; Leasure & Jones, 2008), neurogenesis (Leasure & Jones, 2008), and hippocampal expression of various neural-active molecules (Dishman, 1997; Ploughman et al., 2005). But, either treadmill exercise or wheel running has led to improvements in hippocampus-dependent spatial learning and memory (Liu et al., 2009).
Evidence-based studies on humans and animals show the critical role of sleep in certain types of learning and memory as well as neuronal plasticity (Diekelmann & Born, 2010). A famous hypothesis is that post-training sleep facilitates the consolidation of new information from short-term into long-term memory (Walker & Stickgold, 2004). Another hypothesis states that memory trace reactivation and consolidation might take place during sleep (Lee & Wilson, 2002). Several clinical studies have demonstrated that anxiety symptoms are observed in the absence of periods of sleep (Silva et al., 2004; Vollert et al., 2011). Recent studies have indicated that single night or mild Sleep Deprivation (SD) can cause disruptions in various forms of memory, including procedural, implicit, and working memory in humans (Forest & Godbout, 2000; Yoo, Hu, Gujar, Jolesz, & Walker, 2007). There have been reports that one to five days of SD, before executing a cognitive task, has an impact on behavioral efficiency in rodents (Alvarenga et al., 2004; Silva et al., 2004). In other studies, SD was shown to have deleterious effects on Morris Water Maze (MWM) (Hajali, Sheibani, Esmaeili-Mahani, & Shabani, 2012; Rajizadeh et al., 2018; Salari et al., 2015), open field (Hajali et al., 2012) and novel objective recognition (Binder et al., 2012; Palchykova, Winsky-Sommerer, Meerlo, Dürr, & Tobler, 2006; Rajizadeh et al., 2018). Some studies show that female rats are more vulnerable to deleterious effects of SD than male rats (Hajali et al., 2012).
Female hormones such as steroid hormones, estradiol, and progesterone affect cognitive performance as well as modulating structural, biochemical, and electrical activity in the hippocampus (Brinton, 2009; Foy, Baudry, Akopian, & Thompson, 2010).
The effect of sleep deficiency on cognitive performances has mostly been studied during adulthood in both human and animal studies and very little data exist concerning the effects of poor sleep in gonadal hormone-depleted status (e.g. postmenopausal women or OVX animal models) (Henderson & Sherwin, 2007; Mitchell & Woods, 2011).
Previous publications have demonstrated that forced exercise can ameliorate cognitive impairments followed by SD (Saadati et al., 2015; Salari et al., 2015) and also we showed in a previous study that voluntary exercise improved cognitive impairments due to SD in intact female rats (Rajizadeh et al., 2018). In this study, we evaluated the role of voluntary exercise on cognitive impairments followed by SD in ovariectomized (OVX) adult female rats using behavioral studies.
2. Materials and Methods
2.1. Animals
All experimental protocols were carried out according to the guidelines approved by the Ethics Committee of Kerman Neuroscience Research Center. We tried to reduce the discomfort for the animals during our experiments based on (Ethics Code: KNRC-95-30). Adult female Wistar rats with a weight range of 200-250 g were chosen and divided into seven groups. The animals were put into cages with free access to food and water. The temperature was fixed at 23±1°C and the rats were under 12-12 h light-dark cycle (lights on: 07:00-19:00). A total of 42 Ovariectomized (OVX) rats were assigned to subgroups randomly. The first group was the control group. The OVX group was maintained in home cages, the second group was Sleep-Deprived (SD). The third one was labeled as a wide platform (Sham platform) and it could sleep. A wide platform was utilized to understand the effects of the environment on this group. The fourth group was the exercise group with 4 weeks of voluntary exercise. The fifth and sixth groups were wide platform/exercise and exercise/SD, respectively. The seventh group was a separate group of rats known as the sham ovariectomized (Sham.OVX) group that underwent surgery without removing the ovaries. The aim of having a sham surgery group was to understand the effects of surgical stress. All OVX and sham surgery groups were put under ovariectomy surgical procedure. The number of rats in each group was 7 (Figure 1).
.png)
2.2. Surgical procedures
By applying a mixture of xylazine and ketamine with a dosage of and10 mg/kg, IP xylazine, and 60 mg/kg, IP ketamine, general anesthesia was induced and surgical operations were performed. Under aseptic conditions, both ovaries of the rats were discarded through a small incision in the mid-abdomen. After the operation, all rats were housed for three weeks in the animal room (Ben et al., 2010).
2.3. Exercise protocol
In the wheel exercise group, the rats were put in home cages individually, which was connected to a running wheel (diameter, 20 cm; width, 9 cm) with a duration of 24 h for 4 weeks. The number of revolutions as well as and wheel revolutions was counted. The counting was not dependent on the wheel’s direction. All the rats were given the chance of habituation to the experimental environment and this activity took place 30 min per session in 2 days before starting the exercise protocol. This habituation was to minimize nonspecific stress responses (O’Dell et al., 2007; Sheibani, Rafie, Shahbazi, Naghdi, & Sheikh, 2017). About 30 min after the last exercise session, SD began. In the exercise group, the average exercise in 4 weeks was 21563.82 m among all the rats, and in the exercise/SD group, the average exercise was 25459.96 m.
2.4. Induction of Sleep Deprivation (SD)
A multiple platform device was utilized for inducing SD. The apparatus dimensions were 90×50×50 cm and it also contained 10 columns. The columns were 10 cm high, with 7 cm diameter, and placed 2 cm above the surface of the water, in two rows. These rows were organized 10 cm apart in an edge to edge manner, which gave the rats enough freedom to jump from one platform to another. Because of maintaining social stability, in this method, 4 rats were settled together in the same cage. The rats could freely access food pellet baskets which were always provided as well as clean water bottles. Food pallets and water bottles were positioned on the top of the cage. In this research, the duration of SD was 72 h (Salari et al., 2015). SD paradigm was done 30 min following the last exercise session in the exercise/SD groups in the course of SD interval, with a duration of 72 h at a temperature of 23+1°C. Light/dark cycles were both kept under controlled conditions. The possibility of stress in a novel environment was examined by putting the rats of the control group in a similar chamber with wider platforms. This platform is known as a sham platform which is 10 cm high and 15 cm in diameter. It was designed big enough so the rats could sleep without falling into the water (Hajali,Sheibani, Esmaeili-Mahani, & Shabani, 2012; Rajizadeh et al., 2018).
2.5. Morris Water Maze (MWM)
MWM is a black circular pool with a height of 80 cm and a diameter of 160 cm. The depth of water in the pool is 40 cm. The necessary technology is provided to maintain the water at room temperature. The circular-shaped pool is sectioned into four quadrants of equal size. Each quadrant was marked as N, S, E, and W to show the starting points. A square platform with a 10 cm diameter, positioned in the center of the northeast quadrant. This platform was hidden at 1.5 cm beneath the water surface. The MWM is located in a dimly lit room. Diverse extra geometric images such as circles, squares, or triangles were used as cues. These images were attached to various positions on the walls around the maze and the object positions were kept intact during the whole experimental procedure. A smart video tracking system (Noldus Ethovision® system, version 7, Netherlands) was installed to record the performance, and animals were monitored and tracked on the screen of a computer (Hajali et al., 2012). Also, the temperature of the water was fixed at 25±2°C.
2.6. Spatial learning and memory
In a single training protocol, each rat managed to accomplish three blocks. These blocks were subject to a 30-min rest interval. A block was designed with four consecutive trials which had a duration of 60 s and the inter-trial intervals of 60 s. All of the experiments on the rats were carried out after finishing the SD period. Based on the protocol, the tests could only be done between 9:30 and 12:30 AM. On each trial, starting from one of the four quadrants of the maze, the rats were released into the water in a random manner facing the wall of the pool. Each rat experienced 4 different releasing locations. During the phase of acquisition, the platform location was kept intact and rats were given 60 seconds to swim to the hidden escape platform. After the animals discovered the platform, they were given 20-30 seconds to remain on the platform. Then, they were put in a cage to wait for 20–30 s before the start of the next trial. If a rat didn’t manage to find the platform in 60 s, it was gently led toward the platform by the experimenter and after 20-30 s staying on the platform. The rats were put in an animal cage to wait for 20–30 s before the start of the next trial. The data related to the time and distance of finding the platform were gathered for later analysis. After the last training trial, the memory retention test was performed 24 hours later. The memory test was a single probe trial and was performed to examine the spatial memory in the water maze following sleep deprivation. In this trial, the experimenter removed the platform, and the rat was allowed to swim for 60 seconds. The time and distance percentage spent in the target quadrant (quadrant 4) were analyzed and considered as an indicator of spatial memory retention. After the probe trial, a visible platform test was performed to determine any possibility of SD interference with sensory and motor coordination or motivation. In this stage, the animals were tested for their ability to find a visible platform. The platform was placed 2 cm above the water level and was visible with aluminum foil. It should be mentioned that during the procedure, the experimenter remained blind to the rats’ groups (Rajizadeh et al., 2018; Rajizadeh et al., 2019; Salari et al., 2015). About 160 min after SD, MWM took place and all 3 blocks were accomplished on the same day and the probe test was done 24 h after SD.
2.7. Open Field test
The open field test is performed to evaluate the possible impact of SD on anxiety-like behaviors and locomotion. The square-shaped arena is opaque Plexiglas with the dimensions of 90×90×45 cm. This arena is also sectioned into 16 small squares so that the rats spend time in either central or peripheral squares.
In the current study, the rats were positioned in the middle of the arena. An automated video tracking system (Ethovision, version 7, Noldus Technology, Netherlands) recorded and analyzed their behavior during a 5-min interval. The recorded parameters for each rat were the total distance moved, the total time spent in the center or periphery, speed, and the number of grooming and rearing (Rajizadeh et al., 2018; Vaziri et al., 2015). the open field test was carried out exactly 30 min after SD.
2.8. Novel Object Recognition test
In a 10-min habituation session, all animals were given time to explore a plastic cage (60×60×40 cm). In this phase, no object was presented and the cage was equally illuminated. About 30 minutes after the habituation period, the animals were given the freedom to explore two identical objects placed at the same position inside the box for a total of 5 min (training session). A memory retention ability test was performed 45 min after the training session. During a 3-min test session, the animals were exposed to familiar and novel objects placed in the same position that the objects were in the training phase. However, during the test phase, the location of the new object was pseudo-randomly changed to avoid the natural preference of animals for a certain location. All the presented objects (available in duplicate) were similar in material and size but distinct in shape. After each animal, the objects were cleaned and then air-dried using a 70% alcohol box. The exploration time was defined as the duration of sniffing or touching the object with the nose quantified by a camera. Finally, discrimination ratio (as recognition index) is defined as the ratio of time spent for exploring each object divided by the total time spent for exploring both objects multiplied by 100 in training and test phases (Esmaeilpour, Sheibani, Shabani, & Mirnajafi-Zadeh, 2017; Rajizadeh et al., 2018). The test was carried out approximately 80 min after SD.
2.9. Plasma corticosterone measurement
The effect of voluntary exercise and SD on corticosterone levels was evaluated in all animals. All procedures were carried out between 9:10 and 9:30 AM (30 min after the end of the probe test in MWM). Using CO2, the animals were anesthetized. We took trunk blood using plastic polyethylene tubes on ice containing Na2EDTA as an anticoagulant, immediately after decapitation. The blood was centrifuged at 2600 rpm, for 20 min at a temperature of 4°C. The plasma was collected into micro tubes and refrigerated at −80°C for analyzing the corticosterone levels in all of the samples. The plasma sample analysis was carried out by an ELISA kit which is designed for rats and mice. This kit is specifically for someone who is blind to the treatment of the animals (Rajizadeh et al., 2018; Saadati et al., 2015).
2.10. Data analysis
During the acquisition phase, the time spent and the distance traversed by rats to find the hidden platform in the MWM training were analyzed. The analysis was carried out by a two-way Analysis of Variance (ANOVA) parallel with repeated measures. Through this method, the differences in the learning rates among groups (group and block as the factors) were determined. Data analysis related to the collected data from the MWM probe trials, swim speed, and corticosterone levels, and novel objective and open field data were done by a One-way Analysis of Variance (ANOVA). Tukey’s post hoc multiple comparison test was performed to determine points of a significant difference since statistical significance was seen among groups. The data were expressed as Means±SEM, and P<0.05 was considered statistically significant (Saadati et al., 2015).
3. Results
3.1. Effects of sleep deprivation and voluntary exercise on spatial learning and memory
Animals in all groups managed to learn the platform hidden underwater and this learning was done during the acquisition phase. Declined swimming distance and escape latency across blocks of training is considered as evidence for learning (Figure 2 A & B).
Two- way analysis of ANOVA was performed and it was observed that the distance and escape latency of the Sleep-Deprived group (SD) increased significantly in block 2 (Figure 2A; P<0.001 & Figure 2B; P<0.001) and block 3 (Figure 2A; P<0.05 & Figure 2B; P<0.01) in comparison to the control group. Sleep-deprived rats showed better potential in finding the hidden platform and it was improved significantly in a 4-week running wheel exercise. This was seen by observing the significant decrease in their distance of swimming in block 2 (Figure 2A; P<0.05) and block 3 (Figure 2A; P<0.05) and a significant decline in the escape latency in block 2 (Figure 2B; P<0.05) and block 3 Figure 2B; P<0.01) compared to the SD group in the MWM test.
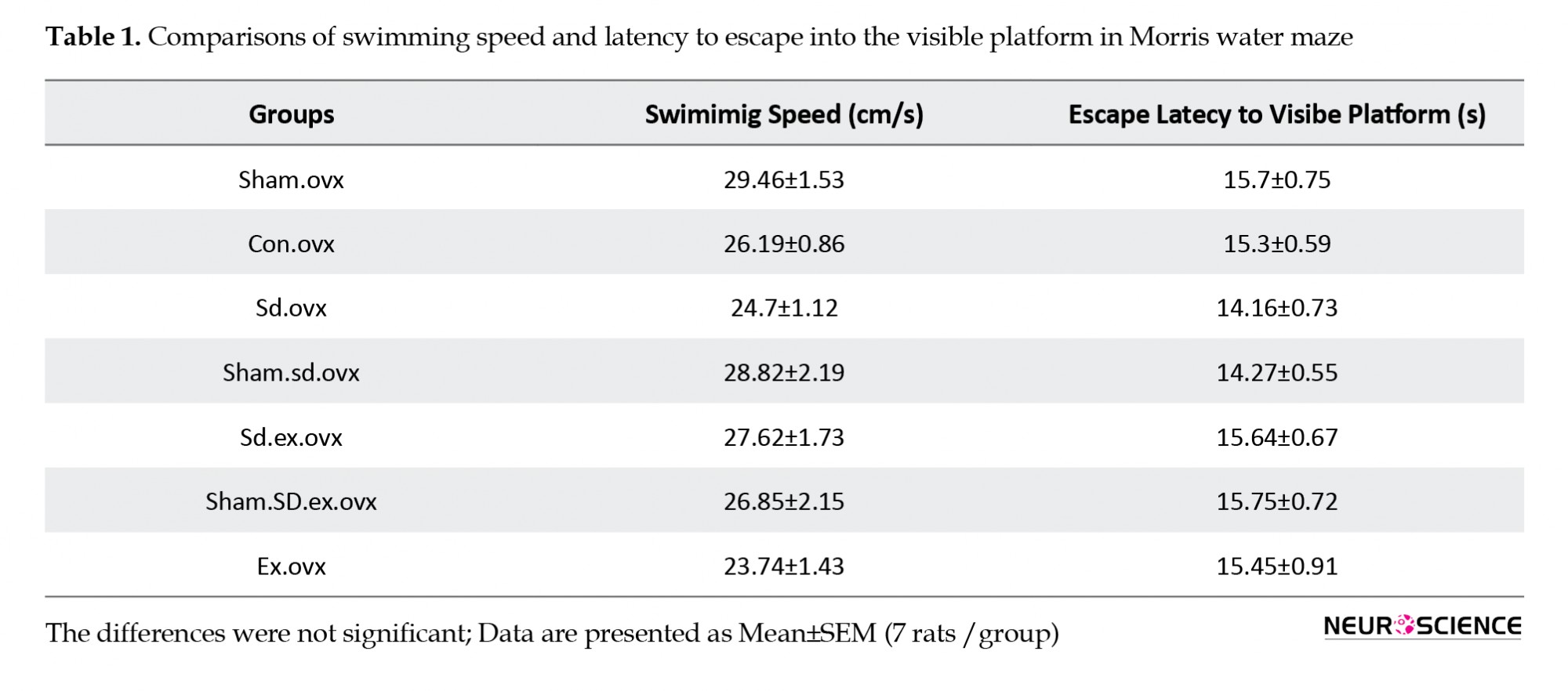
No significant difference was observed in swimming speed and escape latency to find the visible platform among all the groups (Table 1). Therefore, SD did not affect visual and motor function.
.png)
Table. Comparisons of swimming speed and latency to escape onto the visible platform in Morris water maze among groups using one-way ANOVA
Twenty-four hours after the acquisition phase, the probe test was performed to measure long-term retention of spatial memory after sleep deprivation. The obtained results consist of the distance, the number of crossing in the target quadrant, and the mean percentage (%) for time. The probe test results proved less memory retention in sleep-deprived rats. The sleep-deprived rats spent less time and distance in the desired quadrant compared to the control group (Figure 3: P<0.01 for time; Figure 3: P<0.01 for distance and Figure 3: P<0.01 for crossing) which was an indication of long-term memory impairment. However, during 4 weeks of wheel running exercise in the exercise/sleep-deprived groups, this impairment was significantly prevented since more time was spent in the target quadrant and longer distance was traversed in the target quadrant compared to the sleep-deprived rats (Figure 3: P<0.05 for time & Figure 3: P<0.05 for distance and Figure 3: P<0.05 for crossing) (One-way ANOVA followed by Tukey’s test).
.png)
3.2. Effect of sleep deprivation and voluntary exercise on the novel objective recognition test
In the first trial, two familiar objects were presented in the arena and all groups spent a similar amount of time for object exploration and there were no significant differences between all groups (Figure 4). In the second trial, an assessment of object recognition memory was done by replacing the first trial objects with a copy of the original object. The novel object recognition memory was evaluated as the preference for exploring the novel object. In SD rats, significant disruption in new object recognition memory was observed. SD rats devoted less time for investigating the novel objects in comparison with the familiar objects and significant bias was not observed towards the novel objects. In these animals, the discrimination ratio was significantly lower than that in the control group (Figure 4: P<0.05). Four weeks of wheel running exercise helped ameliorate the impairments induced by SD when a novel objective recognition test was implemented (Figure 4: P<0.05) (One-way ANOVA followed by Tukey’s test).
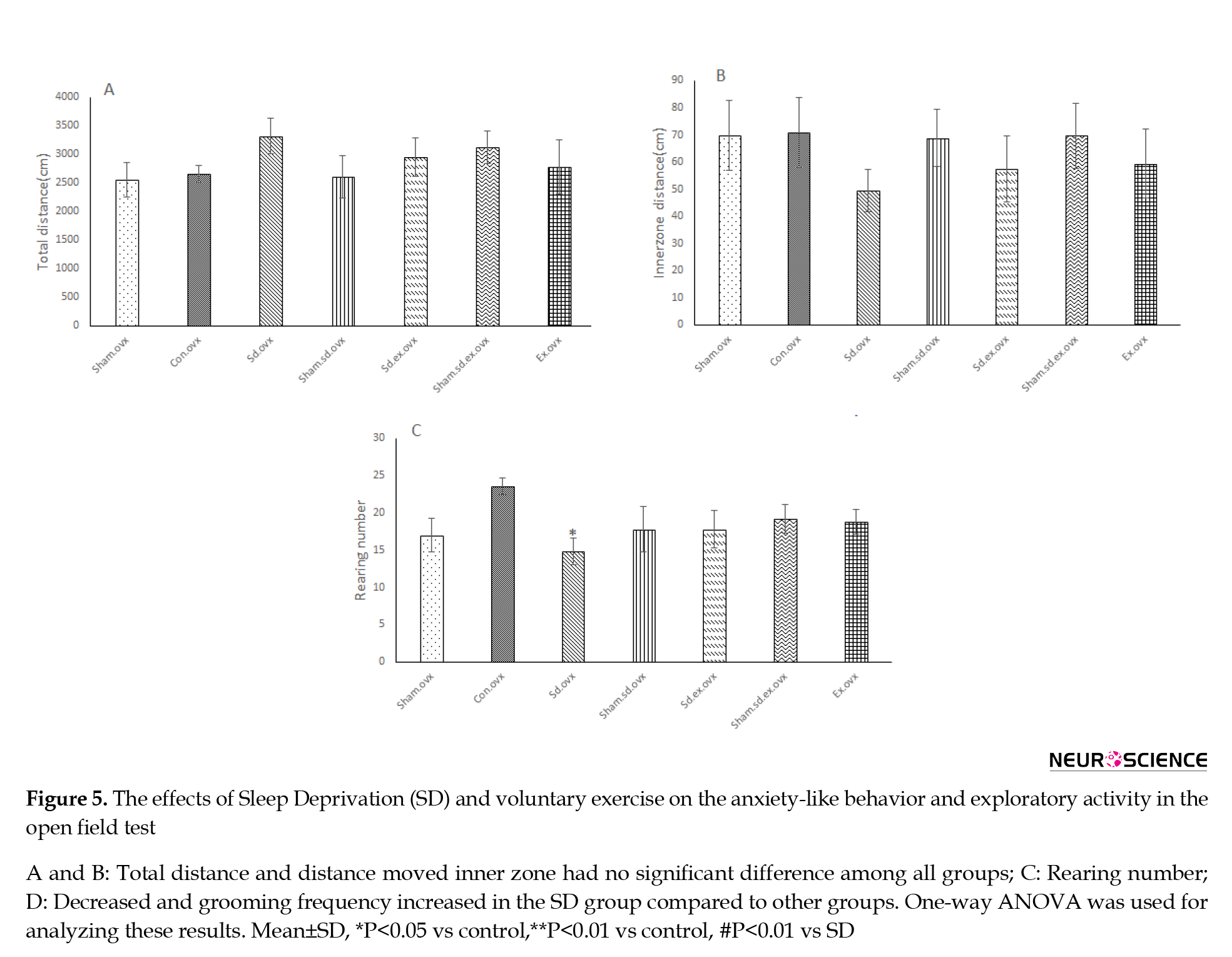
3.3. The effect of sleep deprivation and voluntary exercise on explorative and anxiety-like behaviors
No significant difference in total distance was seen in rats of the SD group in comparison with other groups. No significant differences were seen between all groups in the distance moved in the center in the open field test (Figure 5A & 5B). Rearing numbers were declined significantly in the SD group in comparison with the control group (Figure 5C: P<0.05) and grooming frequency was notably enhanced in the sleep-deprived group compared to the control group (Figure 5D: P<0.01).
Four weeks of wheel running exercise could lead to a rise in the rearing numbers in the sleep-deprived rats but this increase can not be considered as an outstanding value. Also, it significantly decreased the grooming frequency in sleep-deprived rats compared to the sleep-deprived group (Figure 5D: P<0.05) (One-way ANOVA followed by Tukey’s test).
3.4. Effects of Sleep Deprivation (SD) and voluntary exercise on corticosterone level
Our data showed no significant difference in corticosterone levels between all study groups (Figure 6).
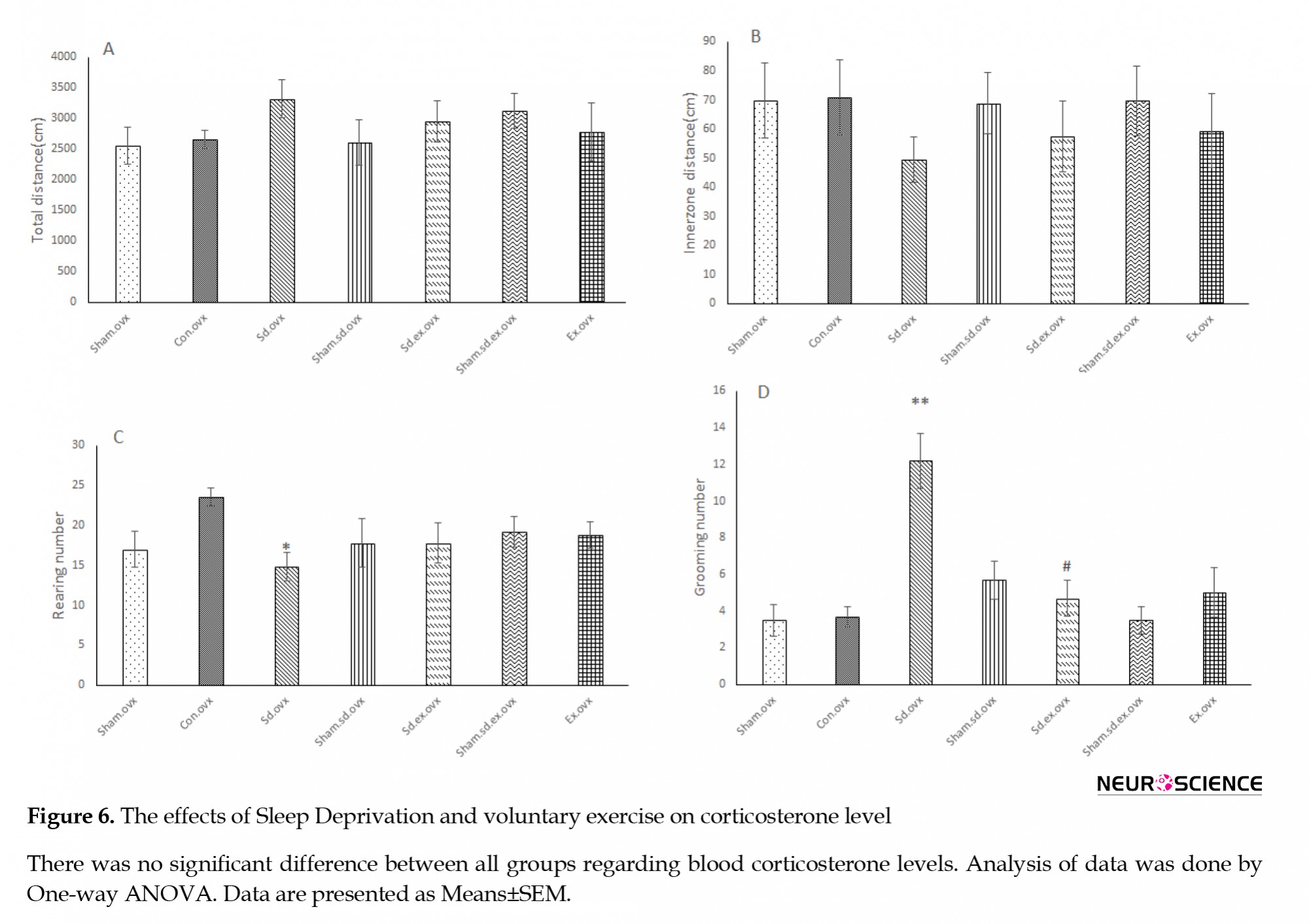
4. Discussion
This study has focused on investigating the impact of wheel running exercise in 4 weeks on learning and memory as well as anxiety-like behaviors followed by 72 h SD in female rats that were ovariectomized. Our results disclosed that SD alone weakens spatial learning in the OVX female rats. It can also disrupt the long-term spatial memory and novel object recognition memory in them. It also seems that voluntary exercise has a protective effect on the OVX rats against the stated negative effects. Also, our results disclosed that SD and voluntary exercise could change anxiety-like behaviors, but these changes were not significantly observed in blood corticosterone levels. In this research, our data revealed that SD had no major effect on voluntary locomotion compared to other groups in OVX female rats.
Sleep deprivation was induced via the multiple platform method. In this method, muscle tone is lost during the period of REM (rapid eye movement) sleep. This technique leads to suppressing about 95% of REM sleep and one can also observe interference with NREM (non-rapid eye movement) sleep (Machado, Hipolide, Benedito-Silva, & Tufik, 2004). Thus, in this research, sleep deprivation in female rats was induced using multiple platform devices. This protocol can omit restraint factors and social isolation. One of these restraint factors is immobilization which is accompanied by other approaches (Suchecki & Tufik, 2000). Also, previous data in our laboratory did not exhibit significant differences between corticosterone levels in SD and exercise groups compared to the control and sham platform (wide platform) groups (Saadati et al., 2015). Besides, the results of the current study indicated that both control and sham platform groups exhibit similarities in spatial memory test (MWM). One can claim that the cognitive impairment in sleep-deprived rats on the narrow platforms was a consequence of sleep loss and not the stress induced by the column in the water apparatus. Besides, the changes observed in the sleep-deprived rats were not a consequence of motor and visual impairments. This claim could be justified by approximately equal swimming velocity and latency to reach the visible platform in our groups.
Evidence supports that learning and memory in the MWM task can be improved by physical exercise (Aguiar et al., 2011; Marlatt, Potter, Lucassen, & van Praag, 2012). Based on evidence from previous research, the beneficial effects of physical exercise on SD-induced memory impairments in male rats can be supported (Zagaar et al., 2012). Exercise can be an ameliorating measure against observed memory impairments in a range of neurodegenerative diseases (Hoveida, Alaei, Oryan, Parivar, & Reisi, 2011). Physical exercise can induce changes in some forms of synaptic plasticity such as LTP (long term potentiation) (Cotman & Berchtold, 2002). Previous data have demonstrated that exercise can alter some neurotransmitters as well as neurotrophic expression in the brain (Cotman & Berchtold, 2002).
Generally, SD negatively affects learning and memory and it seems that exercise plays a protective role for rats against these negative effects (Salari et al., 2015). According to the evidence, one can claim that exercise is one of the potential non-pharmacological interventions that can improve cognitive functions (Shangold, 1990). Furthermore, research has indicated that exercise improves memory function during estrogen deficiency (Ben et al., 2010).
The impact of female sex steroids is clear in regulating sleep in postmenopausal women with low levels of the circulating estrogen (Dzaja et al., 2005; Manber & Armitage, 1999) and they tend to be more vulnerable to the deleterious effects of poor sleep on cognitive performance (Hajali et al., 2012). Albeit, human studies have claimed that differences in sleep, mood, and public health among menopausal and premenopausal women are not significant (Manber & Armitage, 1999). However, various studies on human subjects have reported that postmenopausal women are more vulnerable to deleterious effects of poor sleep on cognitive performance (Dzaja et al., 2005; Manber & Armitage, 1999; Paul, Turek, & Kryger, 2008). Hormone elevations in the ovaries are related to increased learning and memory (Gasbarri, Tavares, Rodrigues, Tomaz, & Pompili, 2012). Furthermore, female rodents in proestrus/estrus performed better compared to females in metestrus and diestrus (van Goethem et al., 2012).
Estrogen treatment is reported to affect memory, especially hippocampus-dependent memory, and this hormone can enhance LTP (Foy, Henderson, Berger, & Thompson, 2000). Many studies have reported that female sex steroid hormones can be a protective agent for a range of neural insults, experimental brain injuries, as well as neurodegenerative diseases (Hogervorst, Williams, Budge, Riedel, & Jolles, 2000; Pike, Carroll, Rosario, & Barron, 2009; Sherwin & Henry, 2008), though the hidden mechanisms underlying this matter is not fully understood (Sherwin & Henry, 2008). In addition, aging female rats with lower estrogen plasma levels in the estropause phase are known to perform worse in a Morris water maze compared to female rats with higher estrogen levels (Hajali et al., 2012). In general, estrogen has been effective in cognitive functions that are complex and time-dependent, including learning and memory processes and these effects can be seen in both animals and humans (Gasbarri et al., 2012). The basal forebrain cholinergic system and hippocampus are the brain systems that may play a role in both attention and memory effects of estrogens (Gibbs & Aggarwal, 1998).
The male rats that experienced SD exhibited significant impairments in learning and memory paradigms such as radial arm water maze (Alhaider, Aleisa, Tran, Alzoubi, & Alkadhi, 2010; Zagaar et al., 2012), MWM (Guan, Peng, & Fang, 2004; Hajali et al., 2012), plus maze discriminative avoidance task (Alvarenga et al., 2008), passive and active avoidance task (Coll-Andreu, Marti-Nicolovius, & Morgado-Bernal, 1991; van Hulzen & Coenen, 1982) and novel object recognition test (Chen, Tian, & Ke, 2014). Furthermore, SD reduces capability for the retention of new information, and disruptions in memory consolidation can be seen (Ravassard et al., 2009; Vecsey et al., 2009). These reports are consistent with our results. Our results in the novel object recognition test showed that SD impaired the ability of rats in distinguishing novel objects and other studies also showed this impairment (Binder et al., 2012; Palchykova et al., 2006). Voluntary exercise in our study could improve this impairment. Other studies confirmed our results (García-Capdevila, Portell-Cortés, Torras-Garcia, Coll-Andreu, & Costa-Miserachs, 2009) but we did not observe any significant effect of only voluntary exercise on novel object recognition. However, some studies have reported the effect of voluntary exercise alone. It seems that the different outcome is due to the duration of exercise (Nichol, Deeny, Seif, Camaclang, & Cotman, 2009; Yuede et al., 2009), novel object recognition test protocol, age, and gender (Hopkins & Bucci, 2010; Hopkins, Nitecki, & Bucci, 2011).
It is believed that deleterious alterations in intracellular signaling molecules, as well as receptors, including NMDA (Chen, Hardy, Zhang, LaHoste, & Bazan, 2006) and AMPA receptors (Hagewoud et al., 2010), lead to the negative effects of SD on learning and memory (Zagaar et al., 2012). Our data demonstrated that SD increased anxiety-like behaviors in the open field test. Other studies revealed that SD could lead to anxiety-like behaviors in an elevated plus maze (Silva, et al., 2004) and open field test (Vollert et al., 2011). Animals that experienced voluntary physical exercise before SD could improve anxiety-like behaviors compared to SD group. Similar studies had demonstrated that forced exercise ameliorated anxiety-like behaviors in sleep-deprived rats (Vollert et al., 2011). Several studies have acquired controversial results by analyzing the relationship between sleep deprivation and anxiety in animal models. Anxiogenic effects were reported in some literature (Vollert et al., 2011) whereas some showed that SD did not exhibit elevated corticosterone level and no change was seen in anxious behavior (Hajali et al., 2012). Furthermore, regular treadmill exercise leads to serum corticosterone level normalization and reduction in anxious behaviors induced by SD (Vollert et al., 2011). Our findings also demonstrated that voluntary exercise prior to SD could reduce anxiety-like behaviors but both of SD and voluntary exercise in this study did not make any significant changes in corticosterone level.
Our data also revealed that SD and voluntary exercise did not exhibit a significant effect on voluntary locomotion in the open field test, which is consistent with other investigations (Hajali et al., 2012).
Based on our findings, removing the ovaries enhances the sensitivity of female rats to the deleterious effects of sleep deprivation on spatial learning compared to the intact female rats. Consistently, in another study, sleep-deprived-OVX female rats exhibited significantly more impairment in learning in the spatial learning task of the MWM test compared to sleep deprived-intact female animals (Hajali et al., 2012). It has been also well documented that female sex steroid hormones can have protective effects against a wide range of neural insults, experimental brain injuries, and neurodegenerative diseases (Hogervorst et al., 2000; Pike, Carroll, Rosario, & Barron, 2009; Scharfman & Maclusky, 2005).
Though the hidden mechanism underlying this process is not well understood (Sherwin & Henry, 2008), some human studies have reported that postmenopausal women are more vulnerable to the harmful effects of poor sleep on cognitive performances (Manber & Armitage, 1999; Paul, Turek, & Kryger, 2008). Our results have revealed that voluntary exercise can have beneficial effects on long-term memory in sleep-deprived OVX female rats. We also showed that voluntary exercise can completely ameliorate sleep deprivation memory decline because memory performance in the SD-exercise groups had reached a similar level compared to the control groups. Other studies proved that voluntary running can induce neurotrophic factors such as Brain-Derived Neurotrophic Factor (BDNF) and fibroblast growth factor in CNS (O’Dell et al., 2007). Voluntary exercise leads to a higher rate of cell proliferation as well as neurogenesis in different locations such as the dentate gyrus in the hippocampus and it also improves learning and memory (Van Praag, Kempermann, & Gage, 1999). The adolescent male and female hippocampus are differentially sensitive to the potentiating effect of voluntary exercise on synaptic plasticity (Titterness, Wiebe, Kwasnica, Keyes, & Christie, 2011). Of course, we neither investigated the role of signaling molecules nor carried out electrophysiological studies in the present study. It seems that further tests are required to examine the effect of voluntary physical exercise on the expression of BDNF mRNA and other signaling molecules and electrophysiological properties in OVX female rats following 72 h SD.
5. Conclusion
In conclusion, the findings of this study revealed that voluntary wheel running exercise can improve SD-induced impairments in spatial learning and memory and recognition memory in the OVX female rats. Also, voluntary exercise could ameliorate anxiety-like behaviors in sleep-deprived OVX female rats although our findings revealed that sleep deprivation and voluntary exercise did not have a significant effect on locomotor activity in OVX female rats.
Ethical Considerations
Compliance with ethical guidelines
There were no ethical considerations to be considered in this research.
Funding
This study was funded by a grant (Grant No.958892) from the National Institute for Medical Research Development (NIMAD).
Authors' contributions
Conceptualization and supervision: Vahid Sheibani and Khadijeh Esmaeilpour; Methodology, investigation, and Writing – review & editing: All authors.
Conflict of interest
The authors declared no conflict of interest.
Acknowledgments
We would like to thank the NIMAD and Kerman Neuroscience Research Center.
References
Aguiar Jr, A. S., Castro, A. A., Moreira, E. L., Glaser, V., Santos, A. R., & Tasca, C. I., et al. (2011). Short bouts of mild-intensity physical exercise improve spatial learning and memory in aging rats: involvement of hippocampal plasticity via AKT, CREB and BDNF signaling. Mechanisms of Ageing and Development, 132(11-12), 560-7. [DOI:10.1016/j.mad.2011.09.005] [PMID]
Alhaider, I. A., Aleisa, A. M., Tran, T. T., Alzoubi, K. H., & Alkadhi, K. A. (2010). Chronic caffeine treatment prevents sleep deprivation-induced impairment of cognitive function and synaptic plasticity. Sleep, 33(4), 437-44. [DOI:10.1093/sleep/33.4.437] [PMID] [PMCID]
Alvarenga, T. A., Patti, C. L., Andersen, M. L., Silva, R. H., Calzavara, M. B., & Lopez, G. B., et al. (2008). Paradoxical sleep deprivation impairs acquisition, consolidation, and retrieval of a discriminative avoidance task in rats. Neurobiology of Learning and Memory, 90(4), 624-32. [DOI:10.1016/j.nlm.2008.07.013] [PMID]
Ben, J., Soares, F. M., Scherer, E. B., Cechetti, F., Netto, C. A., & Wyse, A. T. (2010). Running exercise effects on spatial and avoidance tasks in ovariectomized rats. Neurobiology of Learning and Memory, 94(3), 312-7. [DOI:10.1016/j.nlm.2010.07.003] [PMID]
Binder, S., Baier, P. C., Mölle, M., Inostroza, M., Born, J., & Marshall, L. (2012). Sleep enhances memory consolidation in the hippocampus-dependent object-place recognition task in rats. Neurobiology of Learning and Memory, 97(2), 213-9. [DOI:10.1016/j.nlm.2011.12.004] [PMID]
Brinton, R. D. (2009). Estrogen-induced plasticity from cells to circuits: Predictions for cognitive function. Trends in Pharmacological Sciences, 30(4), 212-22. [DOI:10.1016/j.tips.2008.12.006] [PMID] [PMCID]
Burghardt, P. R., Fulk, L. J., Hand, G. A., & Wilson, M. A. (2004). The effects of chronic treadmill and wheel running on behavior in rats. Brain Research, 1019(1), 84-96. [DOI:10.1016/j.brainres.2004.05.086] [PMID]
Chen, C., Hardy, M., Zhang, J., LaHoste, G. J., & Bazan, N. G. (2006). Altered NMDA receptor trafficking contributes to sleep deprivation-induced hippocampal synaptic and cognitive impairments. Biochemical and Biophysical Research Communications, 340(2), 435-40. [DOI:10.1016/j.bbrc.2005.12.021] [PMID]
Chen, L., Tian, S., & Ke, J. (2014). Rapid eye movement sleep deprivation disrupts consolidation but not reconsolidation of novel object recognition memory in rats. Neuroscience Letters, 563, 12-6. [DOI:10.1016/j.neulet.2014.01.024] [PMID]
Coll-Andreu, M., Marti-Nicolovius, M., & Morgado-Bernal, I. (1991). Facilitation of shuttle-box avoidance by the platform method: temporal effects. Physiology & Behavior, 49(6), 1211-5. [DOI:10.1016/0031-9384(91)90353-P]
Cotman, C. W., & Berchtold, N. C. (2002). Exercise: A behavioral intervention to enhance brain health and plasticity. Trends in Neurosciences, 25(6), 295-301. [DOI:10.1016/S0166-2236(02)02143-4]
Diekelmann, S., & Born, J. (2010). The memory function of sleep. Nature Reviews Neuroscience, 11(2), 114-26. [DOI:10.1038/nrn2762] [PMID]
Dishman, R. K. (1997). Brain monoamines, exercise, and behavioral stress: Animal models. Medicine & Science in Sports & Exercise, 29(1), 63–74. [DOI:10.1097/00005768-199701000-00010] [PMID]
Dzaja, A., Arber, S., Hislop, J., Kerkhofs, M., Kopp, C., Pollmächer, T., & et al. (2005). Women’s sleep in health and disease. Journal of Psychiatric Research, 39(1), 55-76. [DOI:10.1016/j.jpsychires.2004.05.008] [PMID]
Esmaeilpour, K., Sheibani, V., Shabani, M., & Mirnajafi-Zadeh, J. (2017). Effect of low frequency electrical stimulation on seizure-induced short- and long-term impairments in learning and memory in rats. Physiology & Behavior, 168, 112-21. [DOI:10.1016/j.physbeh.2016.11.001] [PMID]
Forest, G., & Godbout, R. (2000). Effects of sleep deprivation on performance and EEG spectral analysis in young adults. Brain and Cognition, 43(1-3), 195-200. https://psycnet.apa.org/record/2000-15775-039
Foy, M. R., Baudry, M., Akopian, G. K., & Thompson, R. F. (2010). Regulation of hippocampal synaptic plasticity by estrogen and progesterone. In Vitamins & Hormones, 82, 219-39. [DOI:10.1016/S0083-6729(10)82012-6]
Foy, M. R., Henderson, V. W., Berger, T. W., & Thompson, R. F. (2000). Estrogen and neural plasticity. Current Directions in Psychological Science, 9(5), 148-52. [DOI:10.1111/1467-8721.00081]
García-Capdevila, S., Portell-Cortés, I., Torras-Garcia, M., Coll-Andreu, M., & Costa-Miserachs, D. (2009). Effects of long-term voluntary exercise on learning and memory processes: dependency of the task and level of exercise. Behavioural Brain Research, 202(2), 162-70. [DOI:10.1016/j.bbr.2009.03.020] [PMID]
Gasbarri, A., Tavares, M. C. H., Rodrigues, R. C., Tomaz, C., & Pompili, A. (2012). Estrogen, cognitive functions and emotion: an overview on humans, non-human primates and rodents in reproductive years. Reviews in the Neurosciences, 23(5-6), 587-606. [DOI:10.1515/revneuro-2012-0051]
Gibbs, R. B., & Aggarwal, P. (1998). Estrogen and basal forebrain cholinergic neurons: Implications for brain aging and Alzheimer’s disease-related cognitive decline. Hormones and Behavior, 34(2), 98-111. [DOI:10.1006/hbeh.1998.1451] [PMID]
Guan, Z., Peng, X., & Fang, J. (2004). Sleep deprivation impairs spatial memory and decreases extracellular signal-regulated kinase phosphorylation in the hippocampus. Brain Research, 1018(1), 38-47. [DOI:10.1016/j.brainres.2004.05.032] [PMID]
Hagewoud, R., Havekes, R., Novati, A., Keijser, J. N., Van der Zee, E. A., & Meerlo, P. (2010). Sleep deprivation impairs spatial working memory and reduces hippocampal AMPA receptor phosphorylation. Journal of Sleep Research, 19(2), 280-8. [DOI:10.1111/j.1365-2869.2009.00799.x] [PMID]
Hajali, V., Sheibani, V., Esmaeili-Mahani, S., & Shabani, M. (2012). Female rats are more susceptible to the deleterious effects of paradoxical sleep deprivation on cognitive performance. Behavioural Brain Research 228(2), 311-18. [DOI:10.1016/j.bbr.2011.12.008] [PMID]
Henderson, V. W., & Sherwin, B. B. (2007). Surgical versus natural menopause: cognitive issues. Menopause, 14(3 Pt 2), 572-79. [DOI:10.1097/gme.0b013e31803df49c] [PMID]
Hogervorst, E., Williams, J., Budge, M., Riedel, W., & Jolles, J. (2000). The nature of the effect of female gonadal hormone replacement therapy on cognitive function in post-menopausal women: A meta-analysis. Neuroscience, 101(3), 485-512. [DOI:10.1016/S0306-4522(00)00410-3]
Hopkins, M. E., & Bucci, D. J. (2010). BDNF expression in perirhinal cortex is associated with exercise-induced improvement in object recognition memory. Neurobiology of Learning and Memory, 94(2), 278-284. [DOI:10.1016/j.nlm.2010.06.006] [PMID] [PMCID]
Hopkins, M. E., Nitecki, R., & Bucci, D. J. (2011). Physical exercise during adolescence versus adulthood: differential effects on object recognition memory and brain-derived neurotrophic factor levels. Neuroscience, 194, 84-94. [DOI:10.1016/j.neuroscience.2011.07.071] [PMID] [PMCID]
Hoveida, R., Alaei, H., Oryan, S., Parivar, K., & Reisi, P. (2011). Treadmill running improves spatial memory in an animal model of Alzheimer’s disease. Behavioural Brain Research, 216(1), 270-4. [DOI:10.1016/j.bbr.2010.08.003] [PMID]
Landers, M. R., Kinney, J. W., Allen, D. N., & van Breukelen, F. (2013). A comparison of voluntary and forced exercise in protecting against behavioral asymmetry in a juvenile hemiparkinsonian rat model. Behavioural Brain Research, 248, 121-28. [DOI:10.1016/j.bbr.2013.04.002] [PMID]
Leasure, J., & Jones, M. (2008). Forced and voluntary exercise differentially affect brain and behavior. Neuroscience, 156(3), 456-65. [DOI:10.1016/j.neuroscience.2008.07.041] [PMID]
Lee, A. K., & Wilson, M. A. (2002). Memory of sequential experience in the hippocampus during slow wave sleep. Neuron, 36(6), 1183-94. [DOI:10.1016/S0896-6273(02)01096-6]
Lin, T. W., Chen, S. J., Huang, T. Y., Chang, C. Y., Chuang, J. I., & Wu, F. S., et al. (2012). Different types of exercise induce differential effects on neuronal adaptations and memory performance. Neurobiology of Learning and Memory, 97(1), 140-7. [DOI: 10.1016/j.nlm.2011.10.006] [PMID]
Liu, Y. F., Chen, H. I., Wu, C. L., Kuo, Y. M., Yu, L., & Huang, A. M., et al. (2009). Differential effects of treadmill running and wheel running on spatial or aversive learning and memory: roles of amygdalar brain-derived neurotrophic factor and synaptotagmin I. The Journal of Physiology, 587(Pt 13), 3221-31. [DOI:10.1113/jphysiol.2009.173088] [PMID] [PMCID]
Machado, R. B., Hipolide, D. C., Benedito-Silva, A. A., & Tufik, S. (2004). Sleep deprivation induced by the modified multiple platform technique: Quantification of sleep loss and recovery. Brain Research, 1004(1-2), 45-51. [DOI:10.1016/j.brainres.2004.01.019] [PMID]
Manber, R., & Armitage, R. (1999). Sex, steroids, and sleep: A review. Sleep, 22(5), 540-541. https://doi.org/10.1093/sleep/22.5.540
Marlatt, M. W., Potter, M. C., Lucassen, P. J., & van Praag, H. (2012). Running throughout middle-age improves memory function, hippocampal neurogenesis, and BDNF levels in female C57BL/6J mice. Developmental Neurobiology, 72(6), 943-52. [DOI:10.1002/dneu.22009] [PMID] [PMCID]
Mitchell, E. S., & Woods, N. F. (2011). Cognitive symptoms during the menopausal transition and early postmenopause. Climacteric, 14(2), 252-61. [DOI:10.3109/13697137.2010.516848] [PMID]
Nichol, K., Deeny, S. P., Seif, J., Camaclang, K., & Cotman, C. W. (2009). Exercise improves cognition and hippocampal plasticity in APOE ε4 mice. Alzheimer’s & Dementia, 5(4), 287-94. [DOI:10.1016/j.jalz.2009.02.006] [PMID] [PMCID]
O’Dell, S. J., Gross, N. B., Fricks, A. N., Casiano, B. D., Nguyen, T. B., & Marshall, J. F. (2007). Running wheel exercise enhances recovery from nigrostriatal dopamine injury without inducing neuroprotection. Neuroscience, 144(3), 1141-51. [DOI:10.1016/j.neuroscience.2006.10.042] [PMID]
Palchykova, S., Winsky-Sommerer, R., Meerlo, P., Dürr, R., & Tobler, I. (2006). Sleep deprivation impairs object recognition in mice. Neurobiology of Learning and Memory, 85(3), 263-71. [DOI:10.1016/j.nlm.2005.11.005] [PMID]
Paul, K. N., Turek, F. W., & Kryger, M. H. (2008). Influence of sex on sleep regulatory mechanisms. Journal of Women’s Health, 17(7), 1201-08. [DOI:10.1089/jwh.2008.0841] [PMID]
Pike, C. J., Carroll, J. C., Rosario, E. R., & Barron, A. M. (2009). Protective actions of sex steroid hormones in Alzheimer’s disease. Frontiers in Neuroendocrinology, 30(2), 239-58. [DOI:10.1016/j.yfrne.2009.04.015] [PMID] [PMCID]
Ploughman, M., Granter-Button, S., Chernenko, G., Tucker, B., Mearow, K., & Corbett, D. (2005). Endurance exercise regimens induce differential effects on brain-derived neurotrophic factor, synapsin-I and insulin-like growth factor I after focal ischemia. Neuroscience, 136(4), 991-1001. [DOI:10.1016/j.neuroscience.2005.08.037] [PMID]
Rajizadeh, M. A., Esmaeilpour, K., Masoumi-Ardakani, Y., Bejeshk, M. A., Shabani, M., & Nakhaee, N., et al. (2018). Voluntary exercise impact on cognitive impairments in sleep-deprived intact female rats. Physiology & Behavior, 188, 58-66. [DOI:10.1016/j.physbeh.2017.12.030] [PMID]
Rajizadeh, M. A., Sheibani, V., Bejeshk, M. A., Mohtashami Borzadaran, F., Saghari, H., & Esmaeilpour, K. (2019). The effects of high intensity exercise on learning and memory impairments followed by combination of sleep deprivation and demyelination induced by etidium bromide. International Journal of Neuroscience, 129(12), 1166-78. [DOI:10.1080/00207454.2019.1640695] [PMID]
Ravassard, P., Pachoud, B., Comte, J. C., Mejia-Perez, C., Scote-Blachon, C., & Gay, N., et al. (2009). Paradoxical (REM) sleep deprivation causes a large and rapidly reversible decrease in long-term potentiation, synaptic transmission, glutamate receptor protein levels, and ERK/MAPK activation in the dorsal hippocampus. Sleep, 32(2), 227-40. [DOI:10.1093/sleep/32.2.227] [PMID] [PMCID]
Saadati, H., Esmaeili-Mahani, S., Esmaeilpour, K., Nazeri, M., Mazhari, S., & Sheibani, V. (2015). Exercise improves learning and memory impairments in sleep deprived female rats. Physiology & Behavior, 138, 285-91. [DOI:10.1016/j.physbeh.2014.10.006] [PMID]
Salari, M., Sheibani, V., Saadati, H., Pourrahimi, A., Esmaeelpour, K., & Khodamoradi, M. (2015). The compensatory effect of regular exercise on long-term memory impairment in sleep deprived female rats. Behavioural Processes, 119, 50-57. [DOI:10.1016/j.beproc.2015.06.014] [PMID]
Scharfman, H. E., & Maclusky, N. J. (2005). Similarities between actions of estrogen and BDNF in the hippocampus: coincidence or clue? Trends Neurosci, 28(2), 79-85. [DOI:10.1016/j.tins.2004.12.005] [PMID]
Shangold, M. M. (1990). Exercise in the menopausal woman. Obstetrics & Gynecology, 75(4 Suppl), 53S-58S. [DOI:10.1097/00006250-199004001-00012]
Sheibani, V., Rafie, F., Shahbazi, M., Naghdi, N., & Sheikh, M. (2017). Comparison of voluntary and forced exercise effects on motor behavior in 6-hydroxydopamine-lesion rat model of Parkinson’s disease. Sport Sciences for Health, 13(1), 203-11. [DOI:10.1007/s11332-017-0354-9]
Sherwin, B. B., & Henry, J. F. (2008). Brain aging modulates the neuroprotective effects of estrogen on selective aspects of cognition in women: A critical review. Frontiers in Neuroendocrinology, 29(1), 88-113. [DOI:10.1016/j.yfrne.2007.08.002] [PMID]
Silva, R. H., Chehin, A. B., Kameda, S. R., Takatsu-Coleman, A. L., Abilio, V. C., & Tufik, S., et al. (2004). Effects of pre- or post-training paradoxical sleep deprivation on two animal models of learning and memory in mice. Neurobiology of Learning and Memory, 82(2), 90-8. [DOI:10.1016/j.nlm.2004.04.005] [PMID]
Silva, R. H., Kameda, S. R., Carvalho, R. C., Takatsu-Coleman, A. L., Niigaki, S. T., & Abilio, V. C., et al. (2004). Anxiogenic effect of sleep deprivation in the elevated plus-maze test in mice. Psychopharmacology (Berl), 176(2), 115-22. [DOI:10.1007/s00213-004-1873-z] [PMID]
Suchecki, D., & Tufik, S. (2000). Social stability attenuates the stress in the modified multiple platform method for paradoxical sleep deprivation in the rat. Physiology & Behavior, 68(3), 309-16. [DOI:10.1016/S0031-9384(99)00181-X]
Titterness, A., Wiebe, E., Kwasnica, A., Keyes, G., & Christie, B. (2011). Voluntary exercise does not enhance long-term potentiation in the adolescent female dentate gyrus. Neuroscience, 183, 25-31. [DOI:10.1016/j.neuroscience.2011.03.050] [PMID]
van Goethem, N. P., Rutten, K., van der Staay, F. J., Jans, L. A., Akkerman, S., & Steinbusch, H. W., et al. (2012). Object recognition testing: rodent species, strains, housing conditions, and estrous cycle. Behavioural Brain Research, 232(2), 323-334. [DOI:10.1016/j.bbr.2012.03.023] [PMID]
van Hulzen, Z. J., & Coenen, A. M. (1982). Effects of paradoxical sleep deprivation on two-way avoidance acquisition. Physiology & Behavior, 29(4), 581-7. [DOI:10.1016/0031-9384(82)90223-2]
Van Praag, H., Kempermann, G., & Gage, F. H. (1999). Running increases cell proliferation and neurogenesis in the adult mouse dentate gyrus. Nature Neuroscience, 2(3), 266-270. [DOI:10.1038/6368] [PMID]
Vaziri, Z., Abbassian, H., Sheibani, V., Haghani, M., Nazeri, M., Aghaei, I., & Shabani, M. (2015). The therapeutic potential of Berberine chloride hydrate against harmaline-induced motor impairments in a rat model of tremor. Neuroscience Letters, 590, 84-90. [DOI:10.1016/j.neulet.2015.01.078] [PMID]
Vecsey, C. G., Baillie, G. S., Jaganath, D., Havekes, R., Daniels, A., & Wimmer, M., et al. (2009). Sleep deprivation impairs cAMP signalling in the hippocampus. Nature, 461(7267), 1122-25. [DOI:10.1038/nature08488] [PMID] [PMCID]
Vollert, C., Zagaar, M., Hovatta, I., Taneja, M., Vu, A., & Dao, A., et al. (2011). Exercise prevents sleep deprivation-associated anxiety-like behavior in rats: Potential role of oxidative stress mechanisms. Behavioural Brain Research, 224(2), 233-40. [DOI:10.1016/j.bbr.2011.05.010] [PMID]
Walker, M. P., & Stickgold, R. (2004). Sleep-dependent learning and memory consolidation. Neuron, 44(1), 121-33. [DOI:10.1016/j.neuron.2004.08.031] [PMID]
Yoo, S. S., Hu, P. T., Gujar, N., Jolesz, F. A., & Walker, M. P. (2007). A deficit in the ability to form new human memories without sleep. Nature Neuroscience, 10(3), 385-92. [DOI:10.1038/nn1851] [PMID]
Yuede, C. M., Zimmerman, S. D., Dong, H., Kling, M. J., Bero, A. W., & Holtzman, D. M., et al. (2009). Effects of voluntary and forced exercise on plaque deposition, hippocampal volume, and behavior in the Tg2576 mouse model of Alzheimer’s disease. Neurobiology of Disease, 35(3), 426-32. [DOI:10.1016/j.nbd.2009.06.002] [PMID] [PMCID]
Zagaar, M., Alhaider, I., Dao, A., Levine, A., Alkarawi, A., Alzubaidy, M., & Alkadhi, K. (2012). The beneficial effects of regular exercise on cognition in REM sleep deprivation: Behavioral, electrophysiological and molecular evidence. Neurobiology of Disease, 45(3), 1153-1162. [DOI:10.1016/j.nbd.2011.12.039] [PMID]